Figures & data
Figure 1. The schematic diagrams of inverse problem domain and light source locations. (a) Illustrates a layout of the inverse problem setting in 2-D. The circular disk Ω corresponds to a horizontal cross-section of the hemisphere part of phantom (the supposed ‘mouse head’ in animal experiments), The computational domain Ω1 is a rectangle containing Ω inside, six light sources are located outside of the computational domain. Because of limitations of our device, we use only three locations of the light source along one line (number 1, 2, 3) to model the source x0 running along the straight line L. Light sources numbered 1, 4, 5, and 6 are used to construct an approximation for the tail function. (b) Depicts the computational domain Ω1 = {(x, z), |x| < 5.83, |z| < 5.83} (unit:mm) and its rectangular meshes for tail functions and inverse calculations for the numerical method of this article. Actual mesh is much dense than these displayed. The diagram is not scaled to actual sizes.
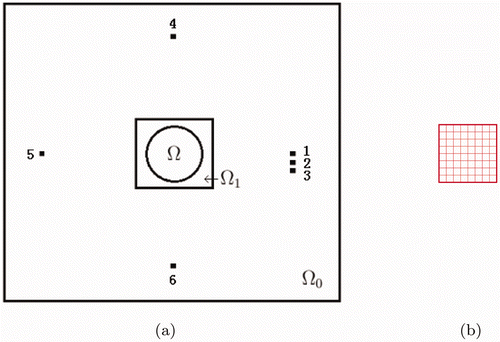
Figure 2. A photograph of the experimental setup. An optical phantom is connected with four laser fibers, the one on the right-hand side is movable to locations 1, 2 and 3 (Figure ). The gelatin made phantom has the shape of the rectangular box with a hemisphere on its top surface. The hemisphere mimics the head of a mouse with a mask exposing the crown part of the mouse. A CCD camera mounted above the phantom (not shown) provides light intensity measurements of the top surface of the phantom.
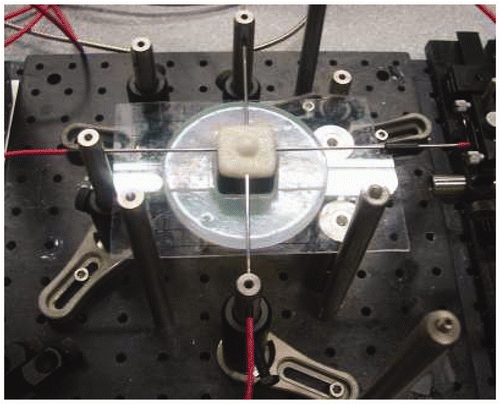
Figure 3. The schematic diagrams of our data acquisition process. (a) schematically depicts a 3-D phantom and its hidden inclusion (shown in meshed surface) 5 mm below the phantom. The light source is placed at various locations on the top surface of the rectangular block (Figure ) and light intensity measurements are taken on the top surface of phantom. (b) The measurement surface by a CCD camera. The data are collected from the surface of the hemisphere as well as from the un-shaded area of top rectangle, both area are lifted from the phantom in this drawing for a better illustrative purpose. The rectangular figure in Figure also illustrates the middle a 2-D cross-section (meshed circle) of the presumed ‘animal head’, at the boundary of which light intensity data are collected for the reconstruction. The light intensity at the 2-D cross-section (except for the boundary) is obstructed by the top surface of the hemisphere. Light sources are also located in the same plane as this cross-section area. The 2-D inverse problem is solved in this cross-section by ignoring the dependence on the orthogonal coordinate.
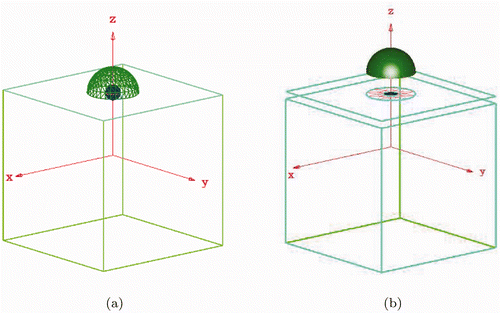
Figure 4. Dimensions of the phantom, shown in a vertical cross-section at the center of the phantom from side view. The circle in Figure corresponds to the boundary of the meshed circle in Figure . This also corresponds to the boundary of the 13 mm diameter circle at a top view above the rectangular box (but not related to circle or semicircle in this graph). The 5 mm diameter hollow sphere is for the placement of inclusions, filled with liquids of different compositions to emulate strokes. The hidden inclusion is 5 mm below the top surface.
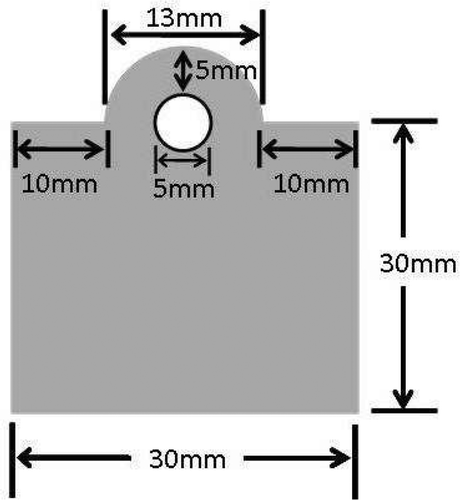
Figure 5. (a) The domain (units: mm) with a triangular mesh. The domain represents a cross-section of our phantom, and the inclusion inside Ω is to be reconstructed. (b) The domain Ω0 = {(x, z), |x| < 23.32, |z| < 23.32} (units: mm). The domain Ω0 contains Ω and light sources. Meshed small square is the domain Ω1 which is the same as on Figure . Computations of the inverse problem are performed in Ω1. (c) Shows Ω0∖Ω the domain used for data processing. To smooth out the measurement noise, Equation (1) with the Dirichlet boundary conditions u ∣∂Ω= ϕ(x, x0),
is solved in Ω0∖Ω. Then the smoothed data along ∂Ω1 is used for the inverse problem.
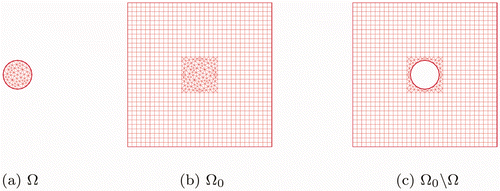
Table 1. Real data.
Figure 6. The function a(x, z) in (49) is depicted. This function was obtained on the first stage procedure for the tail (Subsubsection 5.2.1) as an initial approximation. The initial reconstruction is obtained from a phantom where the theoretical value of the inclusion/background contrast (55) was aincl/ab = 3.
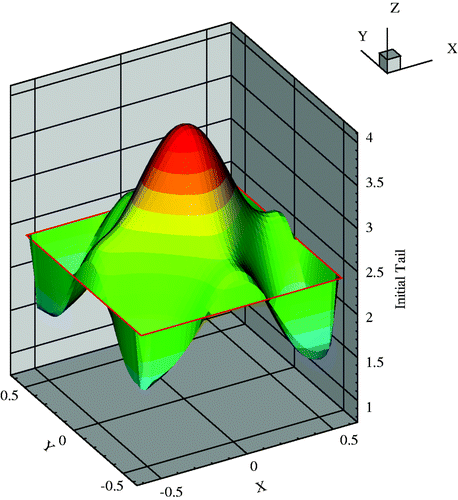
Figure 7. Reconstructed inclusion contrast of data group 1, actual contrasts are 2:1, 3:1, 4:1 and ∞ : 1, from left to right, respectively. The transparent frames show the theoretical values of inclusion/background contrasts of actual inclusions, which are made of different ink-intralipid mix.
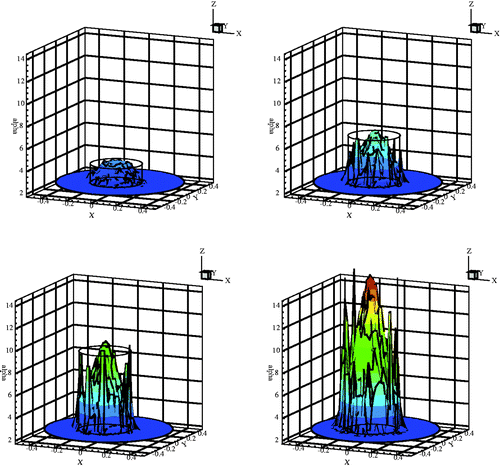
Figure 8. Reconstructed inclusion contrast of data group 2, actual contrasts are 2:1, 3:1, 4:1, from left to right, respectively. The transparent frames show the theoretical values of inclusion/background contrasts of actual inclusions, which are made of different ink-intralipid mix.
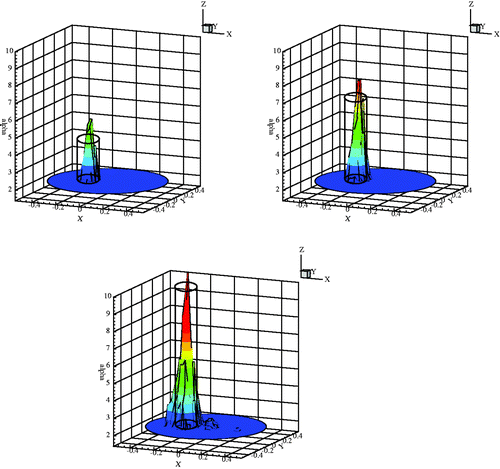
Figure 9. Reconstructed inclusion contrast of data group 3, actual contrasts are 2:1, 3:1, 4:1, from left to right, respectively. The transparent frames show the theoretical values of inclusion/background contrasts of actual inclusions, which are made of different ink-intralipid mix.
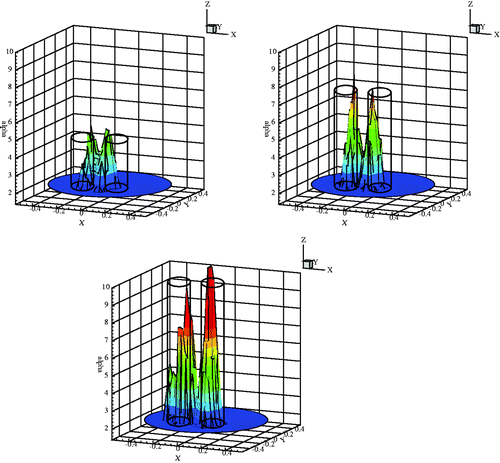