Figures & data
Table 1. Estimated values of parameters for ambient temperature, Ta = 303 K and wind heat transfer coefficient, ha = 25 W/(m2 K).
Figure 3. Comparison of exact and reconstructed heat loss factor distributions; Ta = 303 K (30 °C) and ha = 25 W/(m2 K).
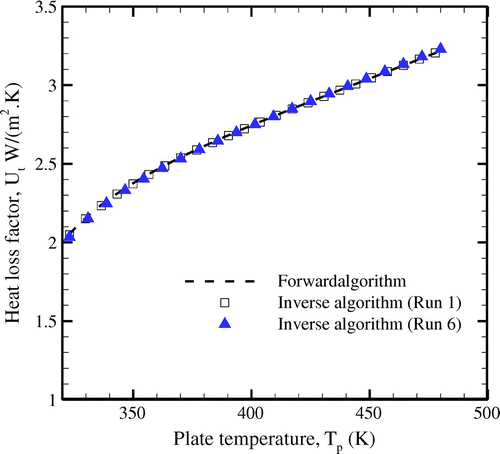
Figure 4. Variation of the objective function with iterations of ABC algorithm (noise-free case); (a) history of 500 iterations, (b) history of first 20 iterations; Ta = 303 K (30 °C) and ha = 25 W/(m2 K).
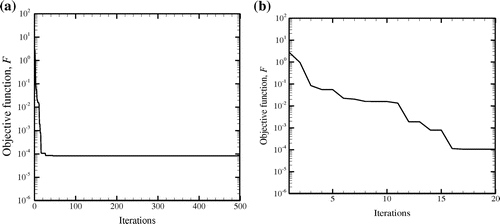
Figure 5. Variation of estimated parameters with iterations of ABC algorithm (noise-free case); Ta = 303 K (30 °C) and ha = 25 W/(m2 K).
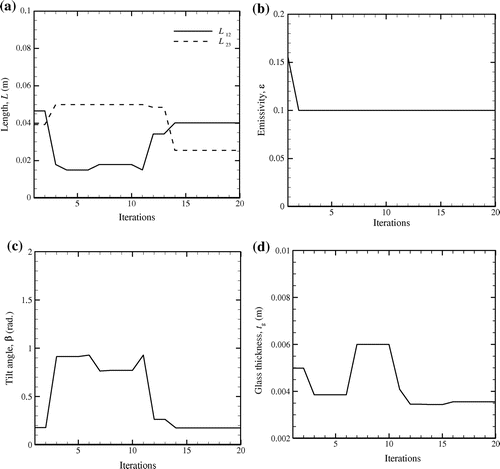
Figure 6. Comparison of exact and reconstructed heat loss factor distributions; , L12 = L23 = 0.025 m, tg = 3 mm, kg = 1.02 W/(m K), Ta = 303 K (30 °C) and ha = 25 W/(m2 K).
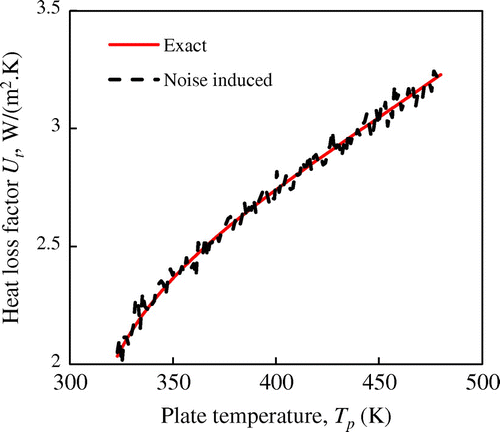
Figure 7. Variation of the objective function with iterations of ABC algorithm (noise-induced case) for Run 6 of Table ; (a) history of 500 iterations, (b) history of first 20 iterations; Ta = 303 K (30 °C) and ha = 25 W/(m2 K).
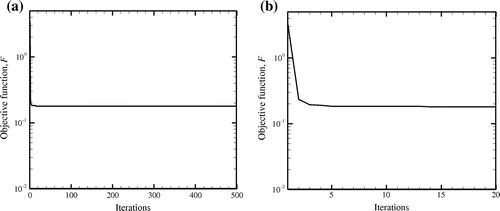
Figure 8. Variation of estimated parameters with iterations of ABC algorithm (noise-induced case) for Run 6 of Table ; Ta = 303 K (30 °C) and ha = 25 W/(m2 K).
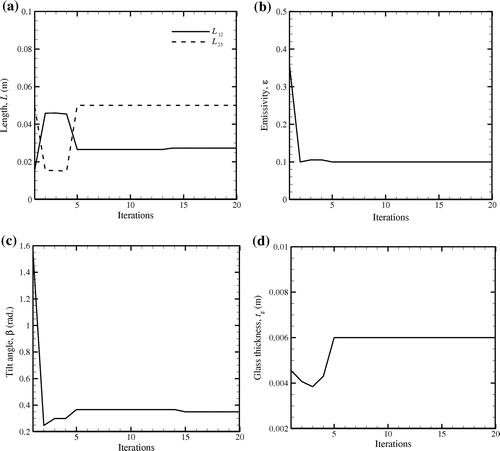
Figure 9. Comparison of sensitivity coefficients of different estimated parameters; , L12 = L23 = 0.025 m, tg = 3 mm, kg = 1.02 W/(m K), Ta = 303 K (30 °C) and ha = 25 W/(m2 K).
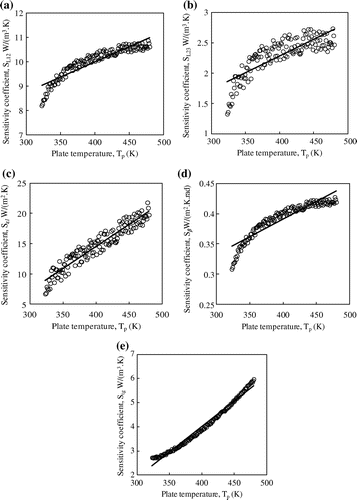
Table 2. Comparison of estimated parameters and objective functions obtained with different optimization methods after 500 iterations.
Figure 10. Comparison of objective functions for different optimization algorithms (a) history of 20 iterations, (b) history of 500 iterations; Ta = 303 K (30 °C) and ha = 25 W/(m2 K).
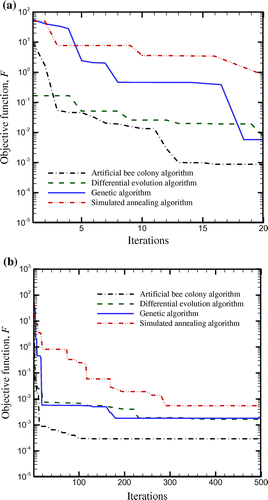
Table 3. Comparison of different optimization methods for 10 additional runs (500 iterations in each case).
Table 4. Estimated values of parameters for variable ambient conditions.
Figure 11. Comparison of objective functions for solar collector operating under varying ambient conditions (a) history of 200 iterations, (b) history of first 20 iterations.
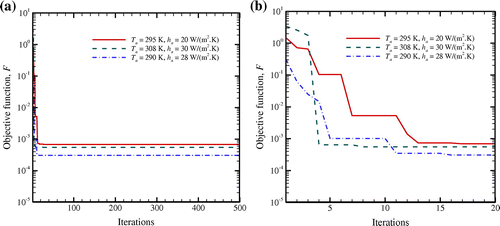
Figure 12. Variation of estimated parameters for ambient temperature, Ta = 295 K (22 °C) and wind heat transfer coefficient, ha = 20 W/(m2 K).
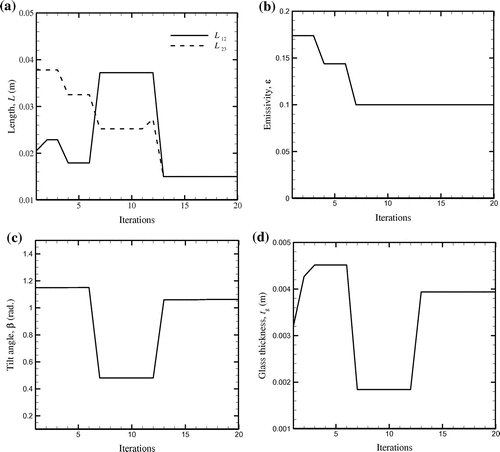
Figure 13. Variation of estimated parameters for ambient temperature, Ta = 308 K (35 °C) and wind heat transfer coefficient, ha = 30 W/(m2 K).
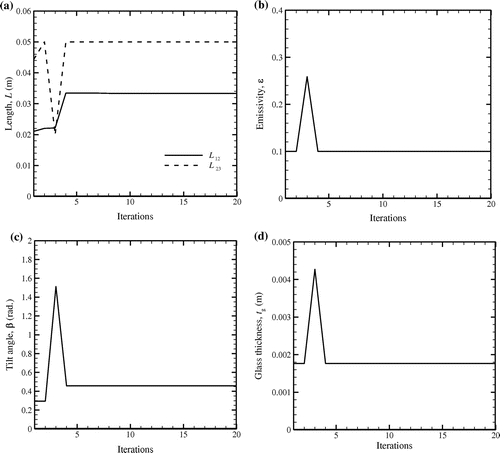