Figures & data
Figure 1. Estimated adsorption isotherms by synthetic data with different error levels of and their corresponding relative errors
. (a)
and
. (b)
and
. (c)
and
. (d)
and
.
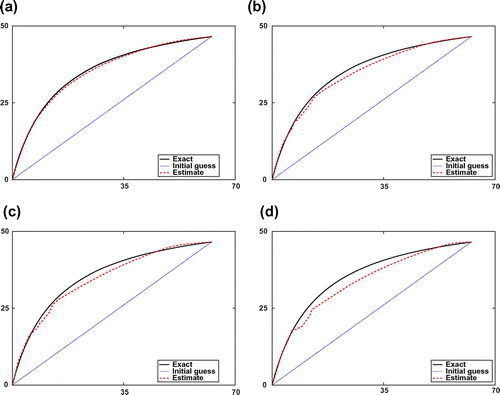
Table 1. Estimated adsorption isotherms and computational costs (the number objective function evaluation (NOFE)) of the boundary fitting method (BF) and the Kohn–Vogelius method (KV) with different error levels for both the Equilibrium-Dispersive (ED) model and the Transport-Dispersive (TD) model with the given mass transfer resistance .
Table 2. Estimated adsorption isotherm parameters and mass transfer resistance by the boundary fitting method (BF) and the Kohn–Vogelius method (KV) with different error levels in the Transport-Dispersive (TD) model.
Figure 2. Numerical results of the boundary fitting method for the Equilibrium-Dispersive model with different error levels at every iteration step l. (a) The value of objective function
and (b) the relative error
of recovered parameters.
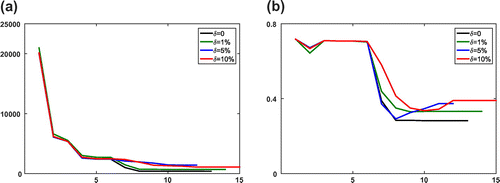
Figure 3. Numerical results of KV method for the Equilibrium-Dispersive model with different error levels at every iteration step l. (a) The value of objective function
and (b) the relative error
of recovered parameters.
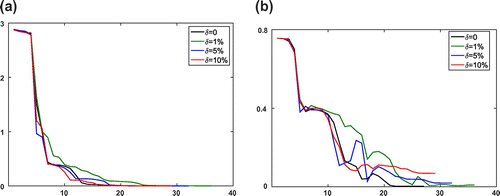
Figure 4. The relative error of estimated adsorption isotherm parameters for the Transport-Dispersive model with different error levels at every iteration step l. (a) The boundary fitting method and (b) the Kohn–Vogelius method.
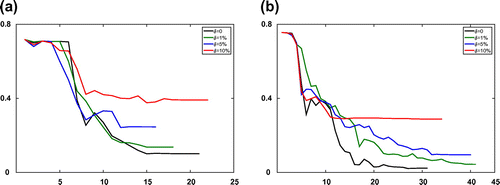
Figure 5. The relative errors of estimated adsorption isotherms and mass transfer resistance for the Transport-Dispersive model with different error levels at every iteration step l. (a) The relative errors of estimated adsorption isotherms
and (b) The relative errors of estimated mass transfer
.
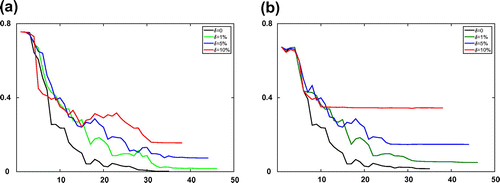
Figure 7. The reconstructed adsorption isotherms by KV method for the Transport-Dispersive model. (a) Fixed mass transfer resistance and
. (b) Estimating mass transfer resistance by inverse algorithm with the constraints
,
.
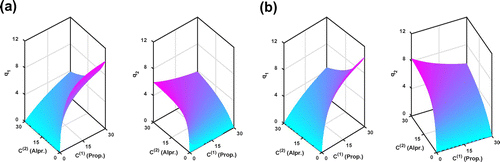