Figures & data
Table 1. The physical and magnetic properties of used material.
Figure 1. The solution of the direct problem for an elliptical defect in three conductive materials, under various strengths of the magnetic field, versus the contour ℓ in degrees.
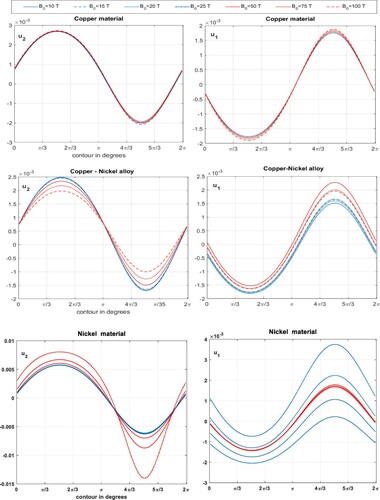
Figure 2. The diffracted surface waves from a defect centred at (1, 1), in three conductive materials, under various strengths of the magnetic field, versus a finite interval on the upper surface.
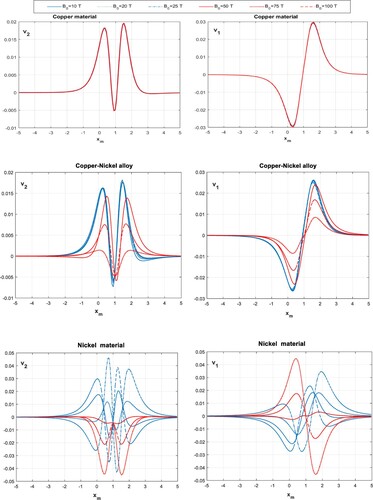
Figure 3. (a) the solution of the direct problem for inclined elliptical defects with three angles in Copper-Nickel material, (b) the solution for smooth rectangular defects with rounded corners in Nickel material, (c) the solution for smooth epicycloidal defects with having different cross-sections.
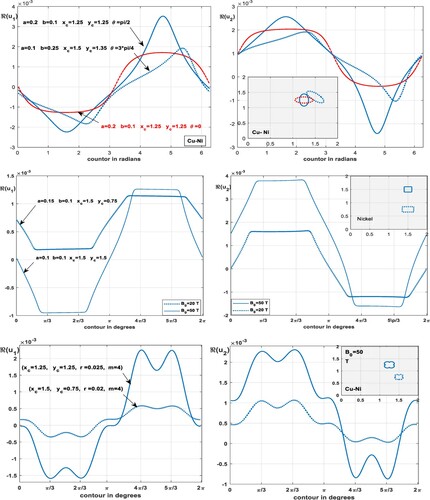
Figure 4. The relative error criterion of ellipse parameters, in three different materials in the absence of the magnetic field, with the corresponding restored vector x of the parameters.
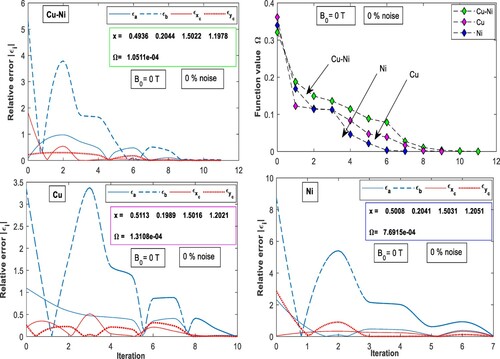
Figure 5. (a) The minimizing path of the discrepancy functional Ω in two noise levels, (b) the relative error criterion of ellipse parameters in a Copper-Nickel alloy, subjected to a magnetic field of strength 50 Tesla, with the corresponding restored vector x of the parameters.
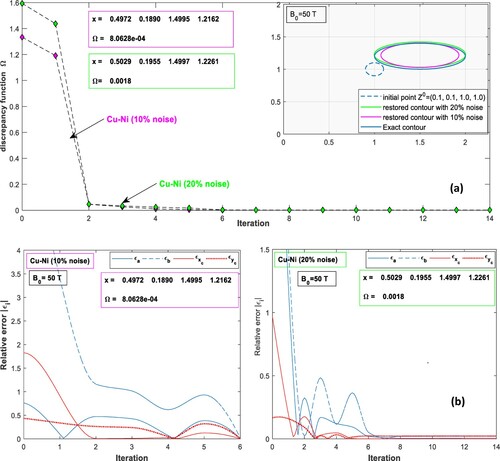
Figure 6. (a) The minimizing path of the discrepancy functional Ω in two noise levels, (b) the relative error criterion of ellipse parameters in Nickel layer, subjected to a magnetic field of strength 50 Tesla, versus the number of iterations, with the corresponding restored vector x of the parameters.
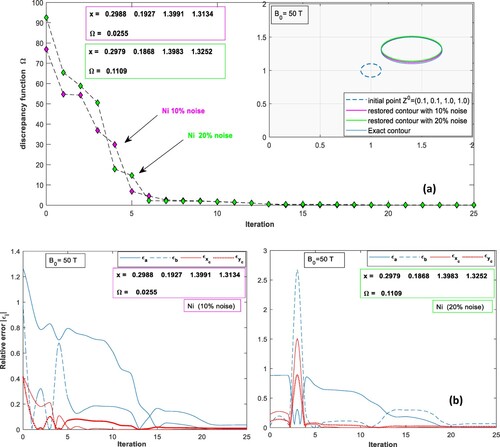
Figure 7. (a) The minimizing path of the discrepancy functional Ω in two noise levels, (b) the relative error criterion of ellipse parameters, close to bottom of a Copper layer subjected to a magnetic field of strength 100 Tesla, with the corresponding restored vector x of the parameters.
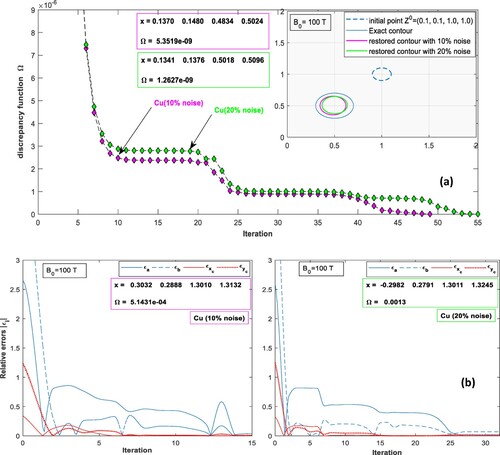
Figure 8. Three noisy levels of minimizing the discrepancy functional Ω, for reconstructing two elliptical defects with inclined angles in a Copper-Nickel layer subjected to an initial magnetic field B
=50 Tesla, with the corresponding restored vector x of the parameters.
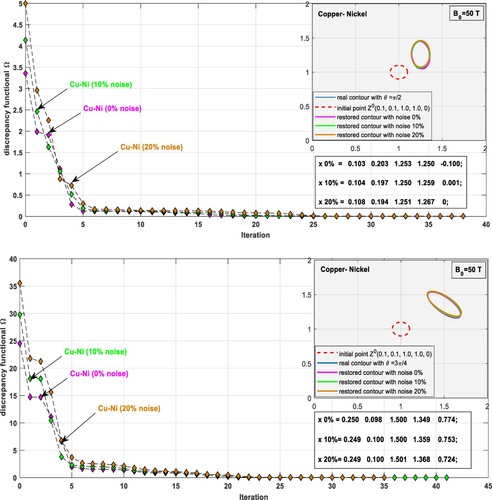
Figure 9. Three noisy levels of the minimizing the discrepancy functional Ω for reconstructing two defects in the shape of a square and rectangle with rounded corners, in a Nickel layer subjected to two different magnetic fields B= 20, 50 Tesla, with the corresponding restored vector x of the parameters.
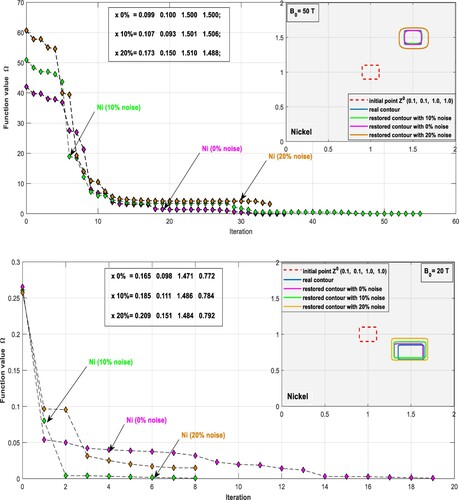
Figure 10. Three noisy levels of minimizing the discrepancy functional Ω for reconstructing two epicycloidal defects, in a Copper-Nickel layer subjected to magnetic field B= 50 Tesla, by using rectangular and elliptical shapes, with the corresponding restored vector x of the parameters.
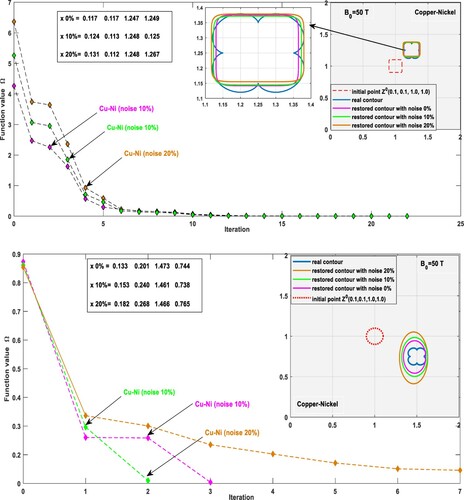
Figure 11. The reconstructing images of an irregular shape with 5 vertices by using elliptical shapes, at different frequencies, in a Copper-Nickel layer subjected to magnetic field B= 10 Tesla.
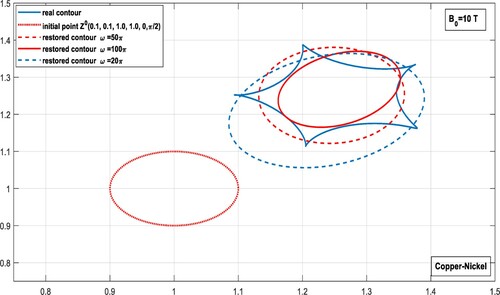
Table 2. Examples of the reconstruction in three different materials, in two noisy cases, under a magnetic field with various strengths versus the relative error criteria.
Table 3. Examples of prediction of the external magnetic field applied upon a copper layer having different defects.