Figures & data
Figure 1. Nanosafety. Together with the biological system, the surface properties and physicochemical identity define the toxic potential of any engineered nanoparticle.
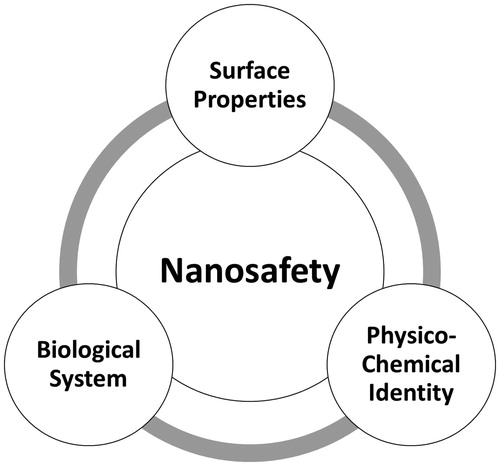
Figure 2. Hazard Evaluation System for Injectable Nanoparticles (HES). The HES hazard evaluation strategy is based on a three-tiered approach, which combines physicochemical characterization of engineered nanoparticles (ENP), ENP interaction, and hazard assessment. This test strategy can be used to (i) determine if a given ENP qualifies as a parentally administered medical application and (ii) identify potential safety issues of injected nanomedicines at early stages of their development. Furthermore, linking the findings from tiers 2 and 3 to the physicochemical properties determined in tier 1 can serve as the basis for in vivo testing strategies, risk assessment strategy set up and future grouping criteria. This will help to design and optimize ENP through safe-by-design principles and support personalized medicine initiatives.
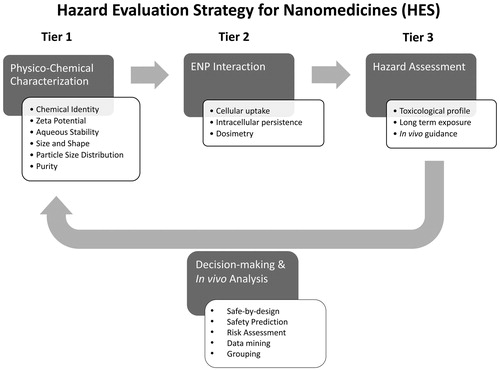
Figure 3. Physico-chemical characterization of nanoparticle properties. The physicochemical characterization of ENP represents tier 1 of the Hazard Evaluation System for Injectable Nanoparticles (HES). After the chemical identity, ζ-potential, and aqueous stability are determined, size, particle size distribution, and purity are examined. In this step-by-step approach, an ENP either moves on through the HES cascade or its safety has to be evaluated separately case-by-case depending on the results obtained during characterization. A candidate passing the entire cascade (Tier 1) qualifies as an injectable nanoparticle according to the HES guideline and moves on to ENP interaction (Tier 2, Figure 4). AR: Aspect Ratio.
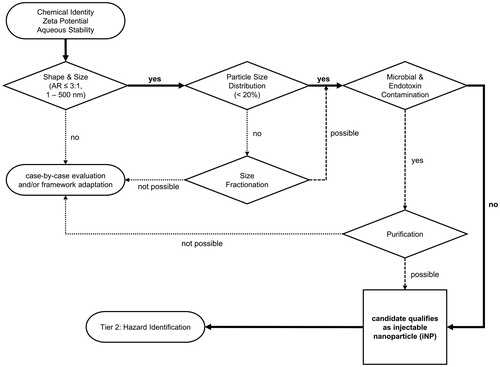
Figure 4. The HES ENP interaction. The HES ENP interaction divides nanoparticles into four different groups. The grouping strategy is based on cellular uptake and intracellular persistence of an iNP. By monitoring these two parameters, distinct hazard assessment strategies can be designed for individual ENP interaction groups. As a general rule, the higher the category the higher the number of possible hazards and thus the number of required safety assessments. It should be noted that the generation of ROS or an involvement and activation of the immune system has a major impact on the interpretation of the HES classification. For example, humoral responses mediated by the complement system may lead to hemolysis and platelet aggregation. Cellular immune responses may lead to particle depletion.
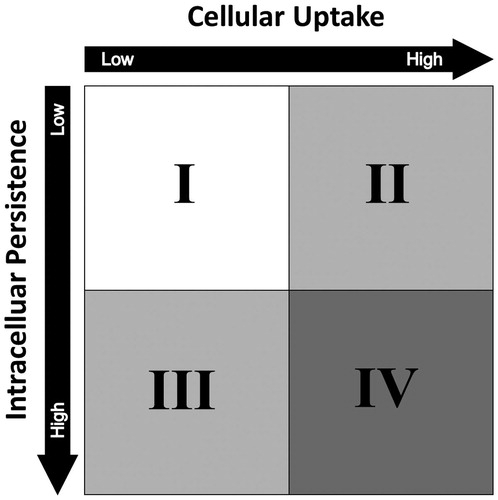
Table 1. Applied doses of FDA approved nanomedicines. Dose, dosing interval, administration route(s), and supplement size of selected FDA approved nanomedicines.
Table 2. Hazard assessment portfolio. This table shows the nine possible parameters that can be determined in the HES Tier 3.
Figure 5. Recommended HES hazard assessments for iNP. Depending on the outcomes in tier 2, an iNP is proposed to pass a hazard assessment cascade individually tailored for one of the four risk categories. Increasing levels of uptake and persistence require more elaborate assay cascades. The eight assays include complement activation, platelet aggregation, hemolysis, oxidative stress, cell viability, phagocytosis, inflammation, and DNA damage.
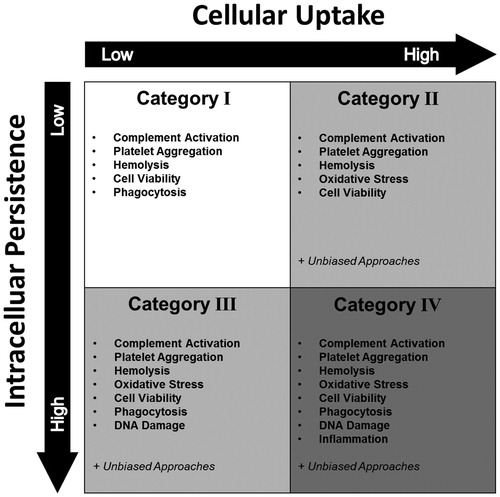
Figure 6. Workflow of a QSAR based grouping and modeling approach. Quantitative structure-activity relationships (QSAR) are used to correlate physicochemical properties of nanoparticles and cytotoxic effects. QSAR model libraries support grouping of ENP.
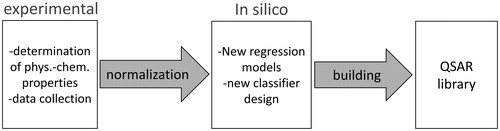
Table 3. Nanoparticle risk assessment based on dose exposure. Starting from a deposited dose, the dose rate or dose equivalents of nanoparticles deposited in specific target tissues or organs are estimated.
Figure 7. Overview of the DF4NanoGrouping approach of ECETOC. The DF4NanoGrouping strategy designed for nanomaterials adopts the Adverse Outcome Pathway (AOP) approach designed for chemicals. Generally, the grouping is divided into four steps where intrinsic material properties, system dependent properties, cellular effects, and apical toxic effects are determined. The experimental part of the concept is a three-tiered assay cascade. Nanomaterials are grouped into soluble, biopersistent, passive or active nanomaterials. The DF4NanoGrouping strategy is not applicable for nanomedicines or nano-therapeutics. It is explicitly designed for the risk assessment of environmental and accidental nanomaterial exposure. Adapted from Arts et al. (2015).
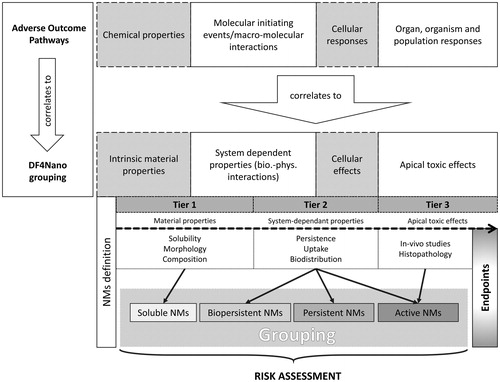
Table 4. Overview of relevant hazard evaluation determinants.