Figures & data
Figure 1. Transmission electron microscopy images of nanocomposites. (a) Commercial nanoparticles mixed in a resin using a planetary mixer – not a desired dispersion due to particle clusters, there are regions with no particles. (b) In-house prepared TiO2 nanoparticles mixed with epoxy using an ex situ method (planetary mixer). In this study this sample is labelled with ex situ nanocomposite – very small particles forming clusters. (c) In situ synthesised particles within a resin (study labelled with in situ nanocomposite) – good dispersion. (d) In situ synthesised particles within polyvinyl alcohol – good dispersion with large irregular particles.
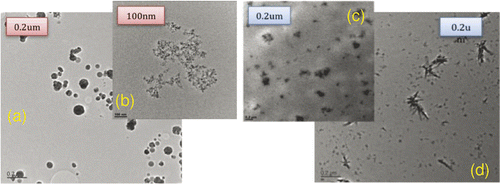
Figure 2. Results of dynamic mechanical analysis, (a) storage and (b) loss moduli, of unfilled resin and two nanocomposites at 1 Hz as a function of temperature.
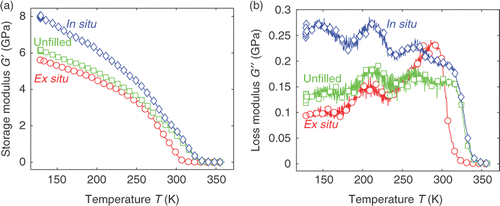
Figure 3. Dielectric susceptibilities (χ = χ ′−ιχ ″) of studied materials, (a) real and (b) imaginary parts.
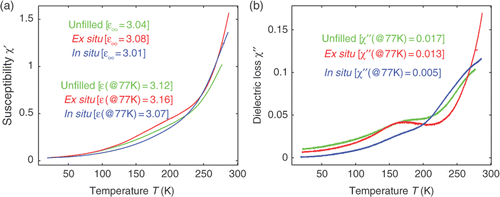
Figure 4. Breakdown statistics analysed with (left) lognormal P L (μ, σ) and (right) two-parameter Weibull P W (α, β) of unfilled resin and its nanocomposites at 77 K. The confidence intervals for each sample are shown for the reader to determine the design parameters for their application. For example, one can consider the percentage probability at 0.1 as the design breakdown strength in engineering applications. The data were collected from dielectric breakdown tests that were performed on multiple samples with thicknesses around 50 µm using a 500 V/s voltage ramp at 77 K.
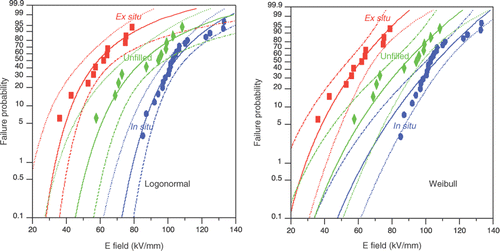