Figures & data
Figure 1. The number of cattle farms in the Bourbonnais region [farms] (dashed line – right axis) and the respective average farm size [ha/farm] (solid line – left axis) (source: Agreste FDS_G_0001).
![Figure 1. The number of cattle farms in the Bourbonnais region [farms] (dashed line – right axis) and the respective average farm size [ha/farm] (solid line – left axis) (source: Agreste FDS_G_0001).](/cms/asset/79d3ab64-6c01-4a13-81d3-de746eb833dd/tjsm_a_2083990_f0001_b.gif)
Figure 2. Illustrative behaviour of a stable system after being affected by a disturbance with a limited duration.
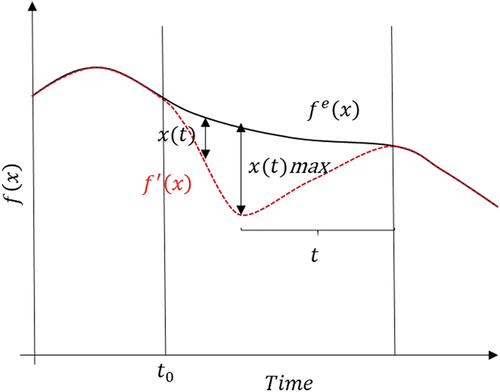
Table 1. Disturbances tested in the model where the disturbance is defined as per EquationEquation (1)
(1)
(1) as the product of the magnitude of the disturbance (M) and its duration (d) and the angle (θ) between the vector components M and d.
Table 2. A summary of the Eur-Agri-SSPs scenarios used in the analysis to explore future trends for the bovine livestock system in the Bourbonnais region and the variables used in the model to generate each scenario.
Figure 3. A causal loop summarising reinforcing loops included in the model representing bovine livestock farms in Bourbonnais.
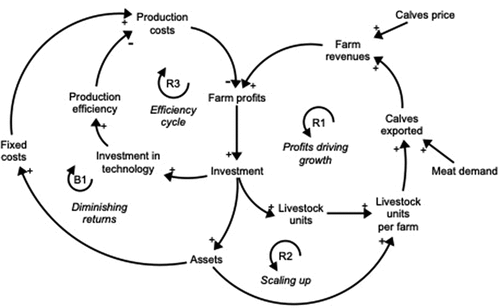
Figure 4. a) A causal loop diagram illustrating the system archetype limits to success and b) an illustrative chart showing the expected relation between farm performance and investment.
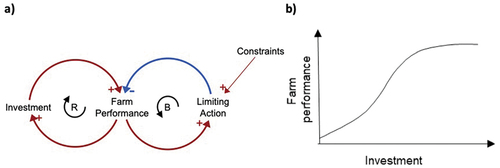
Figure 5. A causal loop summarising some balancing loops included in the model representing bovine livestock farms in Bourbonnais.
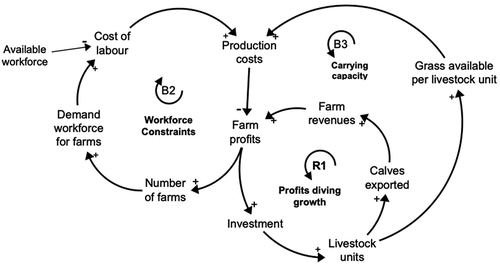
Table 3. Summary of the main inputs and historical data used in the simulation model.
Figure 6. A simplified stock-and-flow diagram showing the main structure included in the simulation model representing livestock farming systems in Bourbonnais. Arrows indicate the direction of causality. The plus (+) in the arrowhead indicates a direct relationship between variables, and the minus (-) indicates an inverse relationship between the variables connected. An “R” has been used to denote reinforcing loops and “B” to denote balancing loops. Boxes represent stocks; arrows with valves represent flows. A stock is the accumulation of the difference between its inflows and outflows. Note that the actual model is more complex than this diagram.
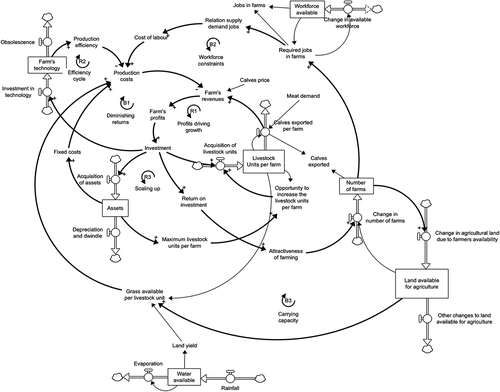
Figure 7. Simulated and historical behaviour for a) average farm size [ha/farm], b) number of farms [farms], c) Jobs in farms [FTE] and d) utilised agriculture area (UAA) [ha] (source for historical behaviour: Agreste FDS_G_0001).
![Figure 7. Simulated and historical behaviour for a) average farm size [ha/farm], b) number of farms [farms], c) Jobs in farms [FTE] and d) utilised agriculture area (UAA) [ha] (source for historical behaviour: Agreste FDS_G_0001).](/cms/asset/23e633da-ebec-485a-a744-4630544bab83/tjsm_a_2083990_f0007_b.gif)
Table 4. Model error for selected variables, where E1 is the error rate and E2 is the error variance.
Figure 8. Simulated behaviour for a) jobs on farms and b) calf exports under the three Eur-Agri-SSPs: Scenario 1 (Sc1): Agriculture on sustainable paths, Scenario 2 (Sc2): Agriculture on established paths, Scenario 3 (Sc3): Agriculture on high-tech paths.
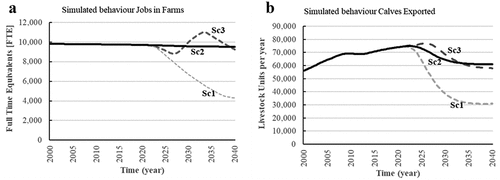
Figure 9. Simulated behaviour for a) jobs on farms and b) calf exports for the disturbances listed in under Eur-Agri-SSPs 2 (Agriculture on established paths).
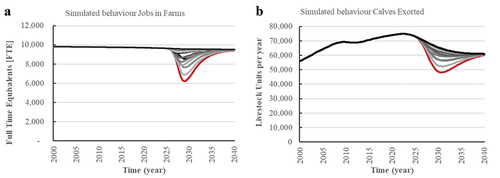
Table 5. Resilience metrics for the disturbances tested in this analysis.
Table A1. Model equations.