Figures & data
Figure 1. Dual system for studying systemic damage responses during tissue regeneration (A) Schematic view of temporal ablation with DtAts. DtAts induces cell death at low temperatures (18°C) and is inactivated at high temperatures (29°C). Thus, by shifting temperatures, a temporal ablation can be induced. With wing disc specific DtAts expression, local tissue injury and subsequent tissue repair can be observed in wing discs. The extent of wing regeneration can be estimated by observing the adult wing phenotype. DtAts: temperature-sensitive form of the diphtheria toxin A domain. (B) Independent tissue-specific gene manipulation by the combination of binary systems. Combination of Gal4/UAS, LexA, or Q system makes it possible to manipulate gene expression in independent tissues. For instance, tissue X specific gene promoter can induce the expression of QF only in tissue X and QF subsequently binds to QUAS, which results in the expression of gene A with no induction of other extrinsic binary system like Gal4/UAS system. Similarly, tissue Y specific gene promoter regulates the expression of gene B through the tissue specific expression of Gal4 and following binding to UAS. (C) Identifying genes required for systemic damage response. Combining a Gal4-based genetic manipulation and a Q-based DtAts ablation, the systemic factors required for disc repair can be investigated in uninjured and remote tissues. Manipulation of different genes in different tissues can be performed by changing the combination of Gal4 drivers or UAS lines. Together with a UAS-RNAi library, this dual system makes it possible to perform genetic screening for studying the tissue interactions involved in tissue repair.
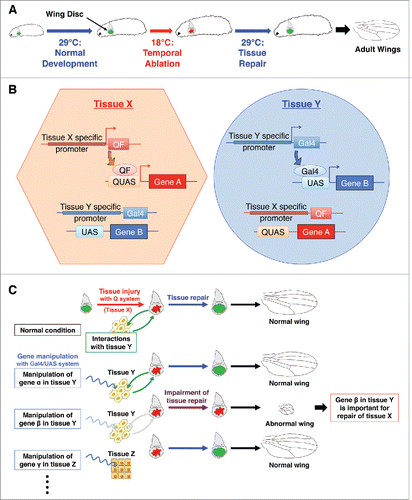
Table 1. Selected examples of SDR in Drosophila.
Figure 2. Systemic damage responses of the fat body methionine metabolism (A) Methionine metabolism in Drosophila. Methionine is converted to SAM by Sams. SAM provides a methyl group for various methyltransferases including Gnmt, and becomes SAH. SAH is further metabolized into homocysteine, which is either regenerated into methionine, or converted into cystathionine through the transsulfuration pathway. SAM also provides an aminopropyl group to synthesize polyamines, such as spermidine and spermine. SAM: S-adenosylmethionine, Sams: SAM synthase, Gnmt: glycine n-methyltransferase, SAH: S-adenosylhomocysteine, CBS: cystathionine β synthase, Ahcy: adenosylhomocysteinases. (B) Enhancement of SAM metabolism in the fat body affects energy wasting and aging. Defects in apoptosis cause necrosis that triggers a systemic immune response including Toll activation in the fat body. dFoxO is activated concomitantly, and induces Gnmt that converts SAM into SAH, possibly functioning as an adaptive response against energy wasting. As aging proceeds, Gnmt is upregulated in the fat body however SAM level is also increased. Enhancing the dFoxO-Gnmt axis rescues age-dependent SAM accumulation and extends the lifespan. (C) Fat body methionine metabolism remotely regulates local tissue repair. Local disc injury non-autonomously affects methionine metabolism in the fat body via an unidentified “Help Me” signal. In turn, appropriate regulation of methionine metabolism in the fat body is required for sufficient tissue repair in damaged discs.
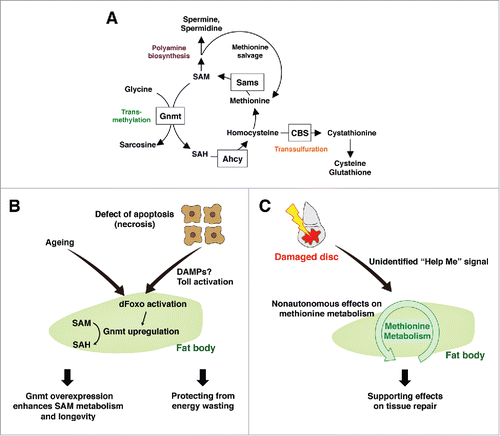