Figures & data
Figure 1. A. Schematic representation of the Ca2+ gradient (adapted from ref. Citation15). The different shades of green are not meant to give an exact representation of differences in Ca2+-concentration. B. Ca2+-induced apoptosis resulting in cell death (adapted from Wikipedia: Apoptosis).L: lysosome, N = nucleus, M = mitochondrion, RER = rough endoplasmic reticulum, SER = smooth endoplasmic reticulum. The red dots with 1, 2, and 3 correspond to the mechanisms 1–3 for keeping [Ca2+]i low (See later).
![Figure 1. A. Schematic representation of the Ca2+ gradient (adapted from ref. Citation15). The different shades of green are not meant to give an exact representation of differences in Ca2+-concentration. B. Ca2+-induced apoptosis resulting in cell death (adapted from Wikipedia: Apoptosis).L: lysosome, N = nucleus, M = mitochondrion, RER = rough endoplasmic reticulum, SER = smooth endoplasmic reticulum. The red dots with 1, 2, and 3 correspond to the mechanisms 1–3 for keeping [Ca2+]i low (See later).](/cms/asset/2d76cc45-2447-47db-a7c7-f5fdc72401e9/kcib_a_1341024_f0001_oc.gif)
Figure 2. Classical view of the major players in the system that regulates intracellular Ca2+ compartmentalization. Cellular Ca2+ import through the plasma membrane occurs largely by receptor-operated (for example, glutamate receptors), voltage-sensitive and store-operated channels. Once inside the cell, Ca2+ can either interact with Ca2+-binding proteins or become sequestered into the endoplasmic reticulum (ER) or mitochondria. The largest Ca2+ store in cells is found in the ER or sarcoplasmic reticulum, with local Ca2+ concentrations reaching millimolar levels. Ca2+ levels in the ER are affected by the relative distribution of sarco(endo)plasmatic reticulum Ca2+-ATPase (SERCA) pumps and of inositol-1, 4, 5-triphosphate (Ins(1, 4, 5)P3) receptors (Ins(1, 4, 5)P3Rs) and ryanodine receptors (RYRs), as well as by the relative abundance of Ca2+-binding proteins (calreticulin, calsequestrin) in the ER or sarcoplasmic reticulum. The cytosolic Ca2+ concentration in unstimulated cells is kept at approximately 100 nM by both uptake into the ER and Ca2+ extrusion into the extracellular space by the plasma-membrane Ca2+-ATPase (PMCA). ER Ca2+ release is triggered by agonist stimulation through the generation of Ins(1, 4, 5)P3 through hydrolysis of phosphatidylinositol-4,5-biphosphate (PtdIns(4,5)P2) operated by a phospholipase C (PLCγ). The mitochondria take up Ca2+ electrophoretically through a uniport transporter and can release it again through 3 different pathways: reversal of the uniporter, Na+/H+-dependent Ca2+ exchange, or as a consequence of permeability transition pore (PTP) opening. The PTP can also flicker to release small amounts of Ca2+. Ca2+ efflux from cells is regulated primarily by the PMCA, which binds calmodulin and has a high affinity for Ca2+. Ca2+ efflux might also be mediated by the Na+/Ca2+ exchanger (NCX). [Ca2+], calcium concentration; DAG, diacylglyceride. This figure was kindly provided by Prof. S. Orrenius. It served as the template for a figure in: Orrenius, S., Zhivotovsky, B., Nicotera, P. (2003). Nature Reviews of Cell Biology 4, 552–556.Citation18 The figure was slightly modified by adding the red dots with FLS, suggesting a role for endogenous sesquiterpenoids (FLS) as agonists for Ca2+-ATPases. Such role is more probable for SERCAs than for PMCAs (hence the question mark).With copyright permission for both the figure and the legend from the publisher and from Prof. S. Orrenius.
![Figure 2. Classical view of the major players in the system that regulates intracellular Ca2+ compartmentalization. Cellular Ca2+ import through the plasma membrane occurs largely by receptor-operated (for example, glutamate receptors), voltage-sensitive and store-operated channels. Once inside the cell, Ca2+ can either interact with Ca2+-binding proteins or become sequestered into the endoplasmic reticulum (ER) or mitochondria. The largest Ca2+ store in cells is found in the ER or sarcoplasmic reticulum, with local Ca2+ concentrations reaching millimolar levels. Ca2+ levels in the ER are affected by the relative distribution of sarco(endo)plasmatic reticulum Ca2+-ATPase (SERCA) pumps and of inositol-1, 4, 5-triphosphate (Ins(1, 4, 5)P3) receptors (Ins(1, 4, 5)P3Rs) and ryanodine receptors (RYRs), as well as by the relative abundance of Ca2+-binding proteins (calreticulin, calsequestrin) in the ER or sarcoplasmic reticulum. The cytosolic Ca2+ concentration in unstimulated cells is kept at approximately 100 nM by both uptake into the ER and Ca2+ extrusion into the extracellular space by the plasma-membrane Ca2+-ATPase (PMCA). ER Ca2+ release is triggered by agonist stimulation through the generation of Ins(1, 4, 5)P3 through hydrolysis of phosphatidylinositol-4,5-biphosphate (PtdIns(4,5)P2) operated by a phospholipase C (PLCγ). The mitochondria take up Ca2+ electrophoretically through a uniport transporter and can release it again through 3 different pathways: reversal of the uniporter, Na+/H+-dependent Ca2+ exchange, or as a consequence of permeability transition pore (PTP) opening. The PTP can also flicker to release small amounts of Ca2+. Ca2+ efflux from cells is regulated primarily by the PMCA, which binds calmodulin and has a high affinity for Ca2+. Ca2+ efflux might also be mediated by the Na+/Ca2+ exchanger (NCX). [Ca2+], calcium concentration; DAG, diacylglyceride. This figure was kindly provided by Prof. S. Orrenius. It served as the template for a figure in: Orrenius, S., Zhivotovsky, B., Nicotera, P. (2003). Nature Reviews of Cell Biology 4, 552–556.Citation18 The figure was slightly modified by adding the red dots with FLS, suggesting a role for endogenous sesquiterpenoids (FLS) as agonists for Ca2+-ATPases. Such role is more probable for SERCAs than for PMCAs (hence the question mark).With copyright permission for both the figure and the legend from the publisher and from Prof. S. Orrenius.](/cms/asset/63a20881-9c63-4445-9898-b36aab3a5a76/kcib_a_1341024_f0002_oc.gif)
Figure 3. Schematic representation of the 2 major models on the mode of action of some lipophilic signaling substances, e.g., Juvenile hormone/FLS and (some) steroids. The classical “Karlson hypothesis” says that such molecules can pass the plasma membrane unhindered, and somehow end up in the nucleus where they act through nuclear receptors/transcription factors. The inbrome modelCitation15 says that lipophilic signaling molecules first enter the whole membrane system and, if they encounter a binding pocket in some of the membrane proteins, they may exert a function. In addition, a second mode of action through nuclear receptors is also a possibility.
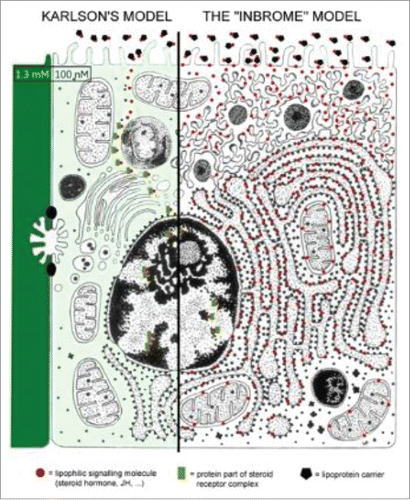
Figure 4. Cartoon depicting the main structural features of the PMCA at resting [Ca2+]i (A) and elevated [Ca2+]i (B). The PMCA consists of an N-terminal domain, 10 transmembrane domains, 4 cytosolic loops and a regulatory C-terminal domain. When [Ca2+]i is elevated, the Ca/CaM binds to the autoinhibitory CaM-binding domain causing a conformational change which exposes the catalytic site which consists the ATP-binding sites and the aspartate residue that is phosphorylated during the reaction cycle. This increases the Ca2+-transporting activity of the PMCA. Reprinted under the Creative Commons Attribution-NonCommercial License from: Bruce J (2013) with thanks.Citation53
![Figure 4. Cartoon depicting the main structural features of the PMCA at resting [Ca2+]i (A) and elevated [Ca2+]i (B). The PMCA consists of an N-terminal domain, 10 transmembrane domains, 4 cytosolic loops and a regulatory C-terminal domain. When [Ca2+]i is elevated, the Ca/CaM binds to the autoinhibitory CaM-binding domain causing a conformational change which exposes the catalytic site which consists the ATP-binding sites and the aspartate residue that is phosphorylated during the reaction cycle. This increases the Ca2+-transporting activity of the PMCA. Reprinted under the Creative Commons Attribution-NonCommercial License from: Bruce J (2013) with thanks.Citation53](/cms/asset/d898825c-0b5b-47ec-b80c-8a6462cb07f2/kcib_a_1341024_f0004_oc.gif)
Figure 5. Schematic representation of the structure of the SERCA2a (10 transmembrane helices) and SERCA2b (11 transmembrane helices) calcium pumps. Slightly modified (by adding a horseshoe-shaped string (in yellow) representing the binding of (endo- or exogenous) sesquiterpenoids linking the TM loops 3, 5 and 7): after Dhitavit et al. in Br J Derm 2004, Vol 150, page 823.Citation55 With permission from the publisher.
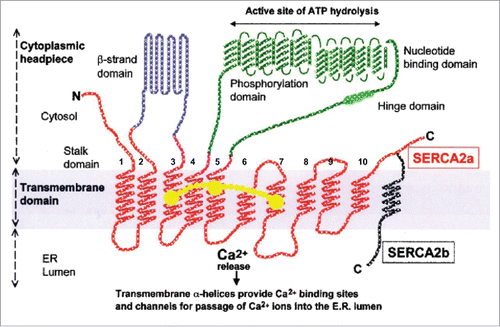
Figure 6. 3-Dimensional view of the SERCA1a and its thapsigargin-binding site (the triangle in A). Thapsigargin (Tg) is a sesquiterpenoid. B. Tilted and enlarged view of the Tg-binding site. From Vandecaetsbeek et al. (2011)Citation16 (Open access). C and D: both farnesol and its ester Juvenile Hormone III are horseshoe-shaped endogenous sesquiterpenoids (From Pubchem: https://pubchem.ncbi.nlm.nih.gov/compound database With thanks).
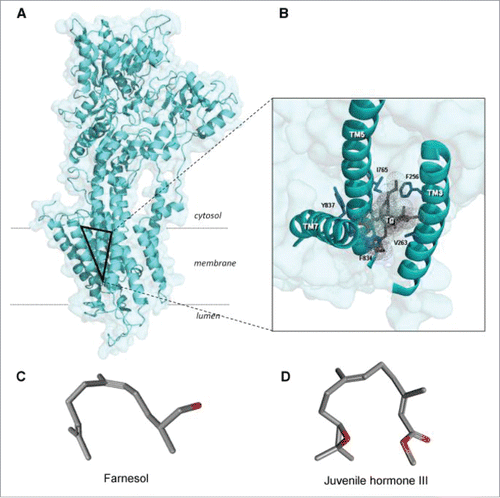
Figure 7. Comparison of the mevalonate biosynthetic pathways in insects and vertebrates. Modified after.Citation15,58,79 Insects do not have the gene coding for squalene synthase. As a result, they cannot synthesize cholesterol by themselves. Some chemical structures of endogenous sesquiterpenoids, retinoic acid and the SERCA-pump blocker thapsigargin (which is also a sesquiterpenoid) are also represented.
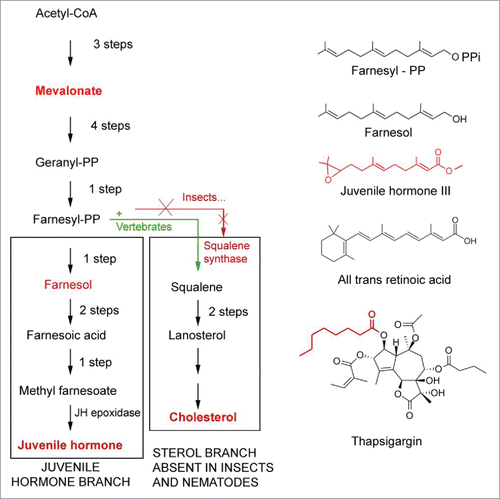
Figure 8. Left: Schematic representation of the structure of bovine rhodopsin (Wikipedia: Rhodopsin).Citation87 It consists of a protein moiety called scotopsin. Like all opsins it has 7 transmembrane domains, and it belongs to the 7TM G protein-coupled receptor family. The 7 TMs form a pocket where a photoreactive chromophore called retinal covalently binds in a very well-defined and evolutionarily well conserved region, namely to a lysine residue in the seventh transmembrane domain. Isomerization of 11-cis-retinal into all-trans-retinal by light induces a conformational change (bleaching) in opsin. Right: 3D-structure of 11-cis-retinal (from Pubchem). For visualization of the flexibility of the molecule, use the “Animate” function in the Pubchem 2–3 D Conformer tool.
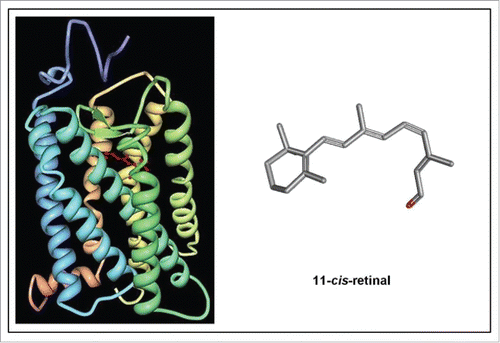
Figure 9. The 2 major molecules instrumental to Prenylation. See also for the horseshoe-shaped configuration of farnesol.
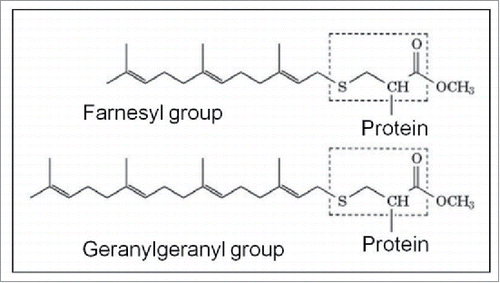
Figure 10. Extremes in longevity: insects vs. patients with the Hutchinson-Gilford Progeria syndrome (modified afterref. 15).
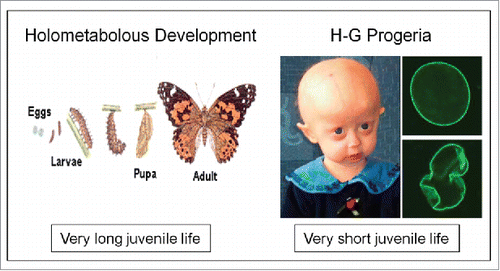
Figure 11. Nuclear lamina (from Wikipedia: Nuclear lamina).Citation97 The localization of lamins just underneath the nuclear envelope is shown in an electron-micrograph and in a schematic representation. For full details, see Wikipedia.
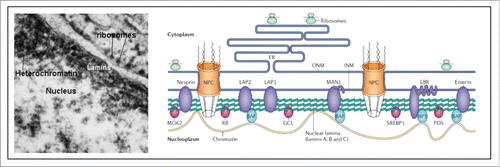