Figures & data
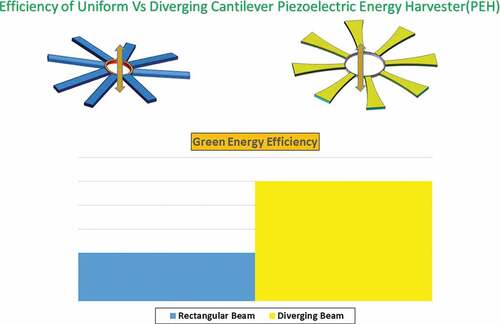
Figure 1. A 3D schematic model of piezoelectric-based cantilever energy harvester with non-uniform width and height.
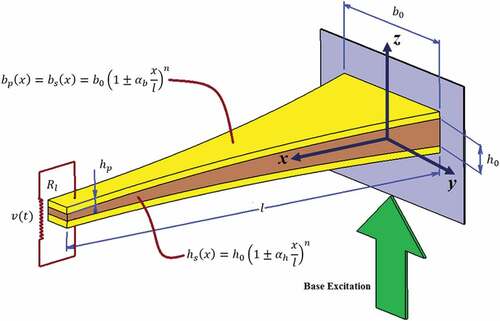
Table 1. Properties of the uniform bimorph piezoelectric cantilever beam.
Table 2. Fundamental resonance frequency of piezoelectric energy harvester without tip mass.
Table 3. Fundamental resonance frequency of piezoelectric energy harvester with tip mass (0.239 g).
Table 4. Properties of the tapered bimorph piezoelectric cantilever beam.
Table 5. Validation of piezoelectric energy harvester’s voltage for different tapering values.
Figure 4. Power output variation versus polynomial degree under different tapering ratios in width direction ().
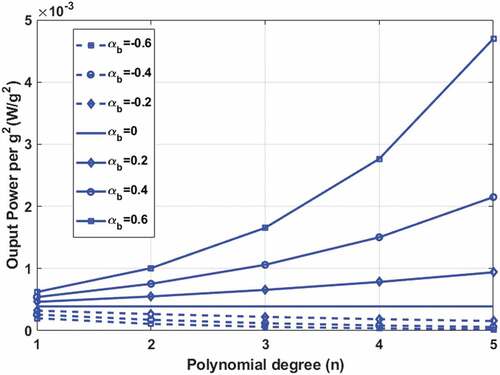
Figure 5. Power output variation versus polynomial degree under different tapering ratios in height direction ().
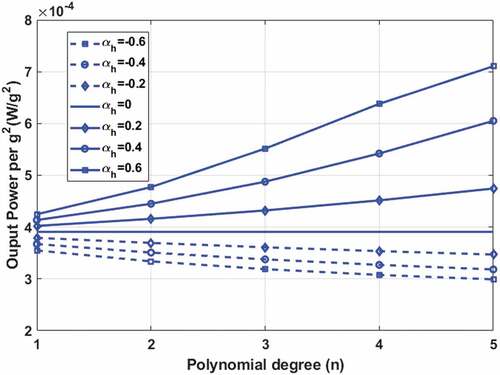
Figure 6. Efficiency variation versus polynomial degree under different tapering ratios in width direction ().
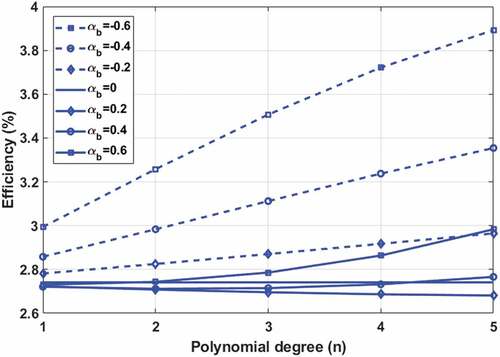
Figure 7. Efficiency variation versus polynomial degree under different tapering ratios in height direction ().
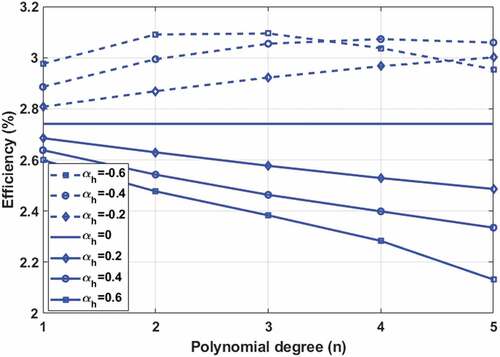
Figure 11. Comparison of power FRFs between one uniform beam and an array of multiple uniform beams.
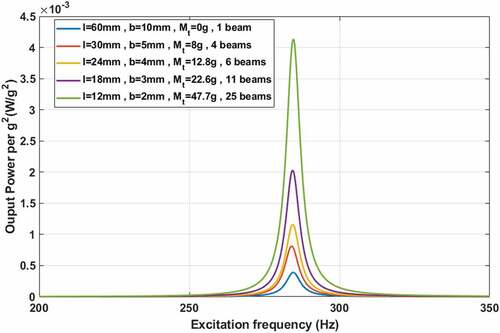