Figures & data
Figure 1. Beijing (China) is comprised of the Beijing Plain in the southeast and mountain in the west and north. The Beijing Plain is clearly delineated with the purple polygon.
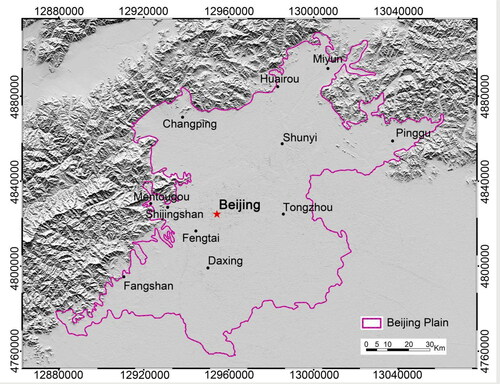
Table 1. Envisat, Radarsat-2 and TerraSAR-X data of Beijing Plain acquired during 2007–2019 and their parameters.
Figure 2. The annual rates of subsidence between 2015 and 2017 are compared with the levelling data. A good agreement is represented by the linear polynomial fits with linear correlation coefficients of R2=0.96 in (a) and R2=0.98 in (b).
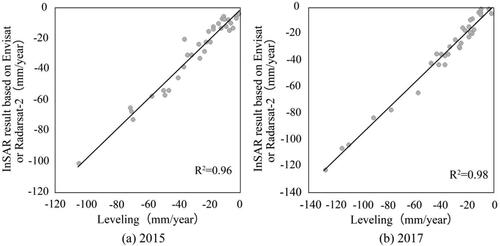
Figure 3. The accumulated subsidence from TerraSAR-X is compared with that from Radarsat-2. the aforementioned subsidence from TerraSAR-X corresponds to the monitoring period between November 2010 and September 2015. However, the accumulated subsidence from Radarsat-2 corresponds to the monitoring period from August 2010 to October 2015. The results are in good agreement with the linear correlation coefficient R2=0.96.
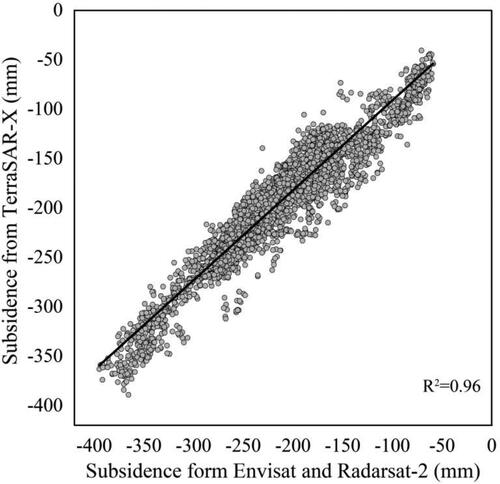
Figure 4. The land cumulative deformation map in the Beijing Plain was acquired by summing 13 mean annual deformation velocities during the period from 2007 to 2019. The map is color-coded from dark blue (represents the short distance between the land and the satellite or land uplift) to dark rose red (denotes the increasing distance between the land and the satellite or land maximum subsidence). This map clearly describes the five subsidence areas, labelled with A, B, C, D and E.
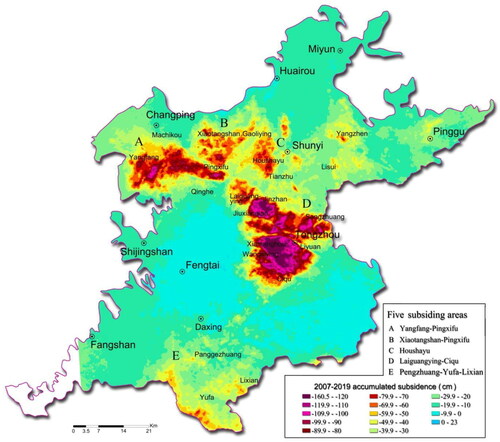
Figure 5. The annual maps of subsidence with rate >5 cm/year show the land subsidence evolution characteristics in Laiguangying–Ciqu (labelled with D in ) during the period between 2007 and 2019. These results are only colour-coded for subsidence rate >5 cm/year to preferably present variation of subsidence bowl (from green [representing subsidence rate between 5 cm/year and 5.9 cm/year] to dark red [denoting subsidence rate between 14 cm/year and 18.8 cm/year]).
![Figure 5. The annual maps of subsidence with rate >5 cm/year show the land subsidence evolution characteristics in Laiguangying–Ciqu (labelled with D in Figure 4) during the period between 2007 and 2019. These results are only colour-coded for subsidence rate >5 cm/year to preferably present variation of subsidence bowl (from green [representing subsidence rate between 5 cm/year and 5.9 cm/year] to dark red [denoting subsidence rate between 14 cm/year and 18.8 cm/year]).](/cms/asset/34e2cde3-7262-4250-b3c5-d2395cec2c83/tgnh_a_1974105_f0005_c.jpg)
Figure 6. (a) Mean annual maximum subsidence rate in Laiguangying–Ciqu (labelled with D in ) during the period from 2007 to 2019; (b) annual subsidence areas (areas with subsidence rate >9 cm/year and between 5 cm/year and 9 cm/year are counted) acquired in the Laiguangying–Ciqu subsidence area by interpolation of those mean annual deformation rates.
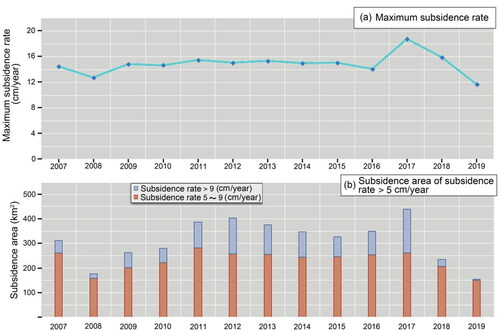
Figure 7. Annual maps of subsidence showing the land subsidence evolution characteristics of the three subsiding areas in northwest of Beijing (labelled with A, B and C in ) during the period between 2007 and 2019. The InSAR acquisition results are all colour-coded from blue (representing positive velocities [land uplift]) to dark red (denoting negative velocities between 14 cm/year and 18.8 cm/year). The northwest subsiding area could be divided into A, B and C subzones, and the three charts below correspond to the annual maximum rates of subsidence in the A, B and C subzones.
![Figure 7. Annual maps of subsidence showing the land subsidence evolution characteristics of the three subsiding areas in northwest of Beijing (labelled with A, B and C in Figure 4) during the period between 2007 and 2019. The InSAR acquisition results are all colour-coded from blue (representing positive velocities [land uplift]) to dark red (denoting negative velocities between 14 cm/year and 18.8 cm/year). The northwest subsiding area could be divided into A, B and C subzones, and the three charts below correspond to the annual maximum rates of subsidence in the A, B and C subzones.](/cms/asset/a9d5e178-d007-4aed-ba98-154dc7ab0c23/tgnh_a_1974105_f0007_c.jpg)
Figure 8. The contrast charts between land subsidence and groundwater depression cone of the Beijing Plain in 2010, 2015 and 2017 demonstrate that the groundwater depression cone locates in the area between Changping and Shunyi. In the Tongzhou (Laiguangying–Ciqu) and Yangfang–Pingxifu subsidence, the groundwater table is basically between 5 and 15 m (Beijing Water Resources Bulletin, 2010, 2015, 2017).
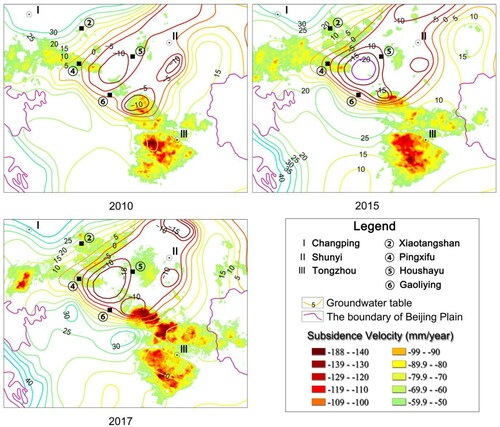
Figure 9. Types of land use and their percentage variation of Laiguangying–Ciqu subsidence in 2010, 2015 and 2017. In the last decade, urban land use extends eastward, and many dry lands converts to urban villages for inhabitant' living.
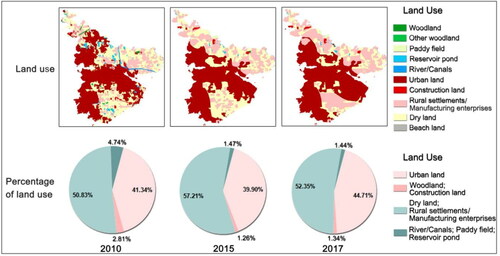
Figure 10. Similar to for northwest subsidence of the Beijing Plain. The yellow colour in A, B and C subzone represents the beach land. We are not sure whether the beach land use needs to exploit groundwater or exploit shallow or deep-confined groundwater.
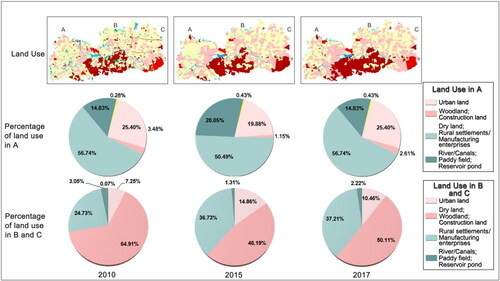
Figure 11. (A) The distribution diagram of two large-scale Alluvial–Proluvial Fan and some small sedimentary depressions in the Late Pleistocene of Quaternary (Cai et al. Citation2009); (B) is the total thickness map of Quaternary compressible layers. (C) and (D) are schematic representations to show geological strata and land deformation along Line AA' and BB', which are both marked by the black lines in (A) and (B) and the two profiles further reveal that land subsidence bowls generally arise in thick multi-layer compressible clay (Cai et al. Citation2009; Lei et al. Citation2016).
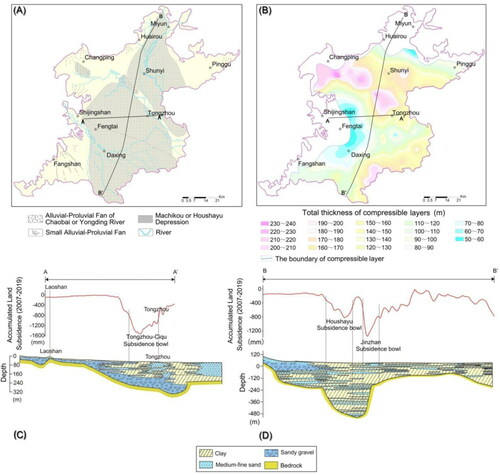
Figure 12. (A) The spatial distribution map between the land subsidence and the pre-existing faults in the Beijing Plain shows subsidence limited by Huangzhuang–Gaoliying, Shunyi–Liangxiang and Sunhe–Nankou Faults, which are active normal-faults. The Huangzhuang–Gaoliying and Shunyi–Liangxiang Faults incline to the South–East, orientating along the North–East direction (Zhao Citation2009; Bai et al. Citation2014; Zhang et al. Citation2016). The striking of Sunhe–Nankou Fault is the North-West and can be divided into two sections, namely, North-West and South-East. The northeast side descends, and the southwest side rises in the South-East section of the Sunhe–Nankou Fault; however, the condition is just on the contrary in the North-West section (Bai et al. Citation2018). (B) The accumulated subsidence map of Beijing Capital Airport during the period between December 2010 and September 2015 more clearly indicates the spatial distribution of the subsiding areas and the spatial relationship with pre-existing faults based on the 3 m resolution TerraSAR-X images.
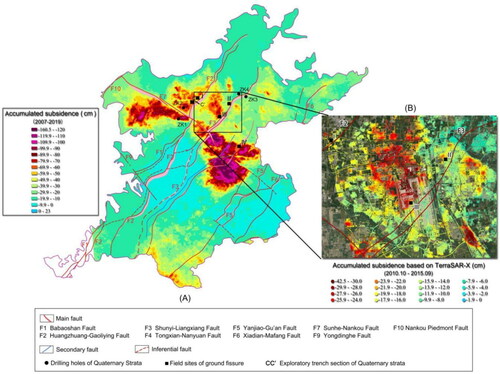
Figure 13. (A) Quaternary stratum histogram. ZK1, ZK2, ZK3 and ZK4 are at both sides of the Sunhe–Nankou and Shunyi–Liangxiang Faults in (Bai et al. Citation2014, Citation2018). The quaternary sediments in ZK2 and ZK3 are much thicker than those in ZK1 and ZK4. (B) Exploratory trench section map along profile CC′ perpendicular to Huangzhuang–Gaoliying Fault in (Zhang et al. Citation2016).
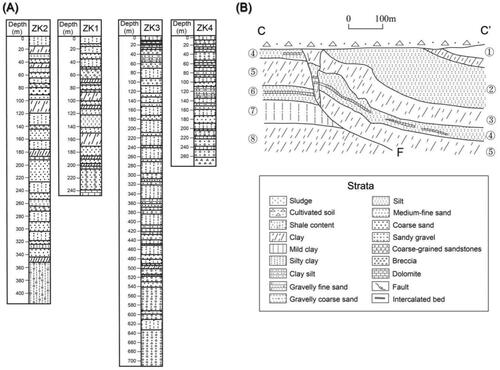
Figure 14. This map shows several field sites located in I, II, III and IV (labelled with black square in ). I and IV are ground fissure monitoring stations located in the Xiwang Road, Shunyi District and Songzhuang, Tongzhou District, and they have showed continuous development of ground fissures in recent years. II and III are ground surface rupture phenomena along the Shunyi–Liangxiang Fault (F3). The ground fissure of II occurred near Risheng Building Materials City, Shunyi District and was recorded in August 2020. The ground fissure of III occurred in the south of Terminal 2 of Beijing Capital Airport in 2017, and it has disappeared due to the sealing work of airport pavement.
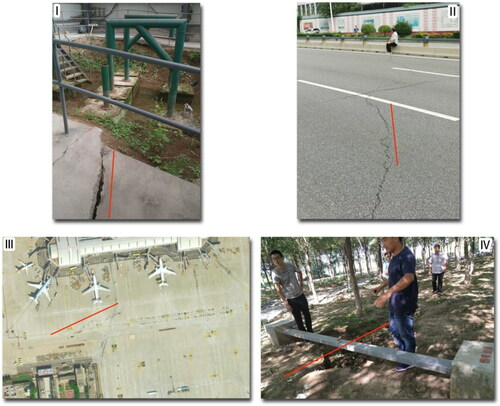