Figures & data
Figure 1. The gastrointestinal system and the role of bile salts in nutrient absorption. Bile salts activate pancreatic lipase producing monoglycerides and free fatty acids. Bile salts aid in the solubilization and absorption of lipids, cholesterol and fat-soluble vitamins by forming mixed micelles. The capital letters A-D indicate duodenum, jejunum, ileum and colon, respectively.
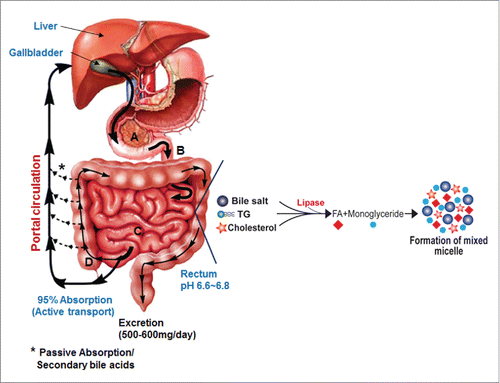
Figure 2. Synthesis of primary bile acids in the liver and biotransformations of bile acids carried out by gut bacteria. In humans, the liver synthesizes cholic acid and chenodeoxycholic acid from cholesterol. The major quantitative and irreversible reactions carried out by gut bacteria include the hydrolysis of conjugated bile acids by BSH and 7α-dehydroxylation of cholic acid and chenodeoxycholic acid. More than 20 bile acid metabolites can be produced from primary bile acids by the gut microbiota. Abb. BSH, bile salt hydrolase; 3α-HSDH, 3α-hydroxysteroid dehydrogenase; 3β-HSDH, 3β-hydroxysteroid dehydrogenase; 7α-HSDH, 7α-hydroxysteroid dehydrogenase; 7β-HSDH, 7β-hydroxysteroid dehydrogenase; 12α-HSDH, 12α-hydroxysteroid dehydrogenase; 12β-HSDH, 12β-hydroxysteroid dehydrogenase.
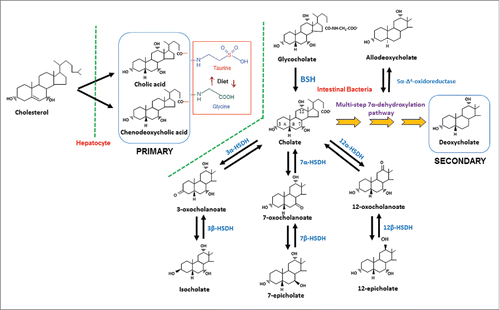
Figure 3. Proposed bile acid 7α-dehydroxylation pathway of cholic acid in Clostridium scindens. The multistep 7α-dehydroxylation pathway converts the primary bile acids cholic acid and chenodeoxycholic acid into the secondary bile acids deoxycholic acid and lithocholic acid, respectively. Abb. baiG, primary bile acid transporter; baiB, bile acid-coenzyme A ligase; baiA, 3α-hydroxysteroid dehydrogenase; baiCD, 7α-hydroxy-3-oxo-Δ4-cholenoic acid oxidoreductase; baiF, bile acid coenzyme A transferase/hydrolase; baiE, bile acid 7α-dehydratase.
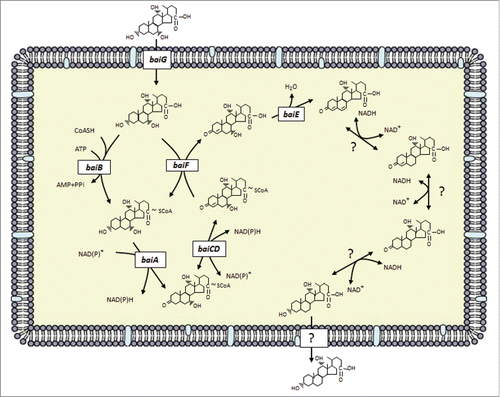
Figure 4. Arrangement of genes in the bile acid inducible (bai) operon in various species of bile acid 7α/β-dehydroxylating gut bacteria. The genes encoding enzymes carrying out bile acid metabolism in gut bacteria capable of producing secondary bile acids. Biochemical pathway leading to secondary bile acid formation is shown in . Amino acid accession numbers are as follows: C. scindens ATCC 35704 baiABCDEAFGHI (EDS05761-EDS05768), baiA2 (EDS06021), 7α-HSDH (EDS05904); C. scindens VPI 12708 baiBCDEA2FGHI (AAC45410-AAC45418), baiJKL (ACF20978-ACF20980), baiA1 (P07914), 7α-HSDH(AAB61151); C. hiranonis TO-931 (DSM 13275) baiBCDEA2FG (AAF22844-AAF22849), baiH (ABQ12723), baiJ (WP_006439754) 7α-HSDH (WP_006440226; C. sordellii VPI 9048 baiCD, baiA2 (EPZ56913, EPZ56915), baiH, baiE (EPZ56916, EPZ56912), 7α-HSDH (EPZ54454); C. hylemonae TN271 baiBCDEA2FGHI (ACF20984-ACF20990), baiJKL (ACF20981-ACF20983) baiA1 (WP_006441384), 7α-HSDH (WP_040435027).
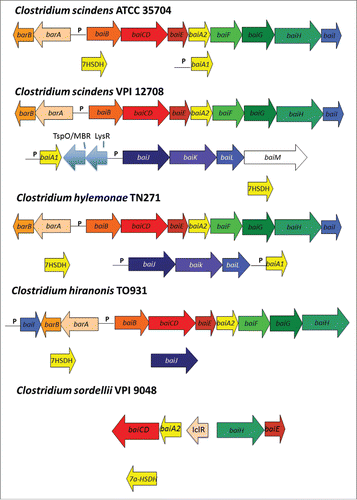
Figure 5. Binding of substrate to bile acid 7α-dehydratase from Clostridium scindens. (A) Predicted binding mode of 3-oxo-Δ4-chenodeoxylcholyl-CoA (3-oxo-Δ4-CDCA-CoA) in the crystal structure of this enzyme. (B) Observed binding of the product, 3-oxo-Δ4,6-lithocholyl-CoA (3-oxo-Δ4,6-LCA-CoA), in product bound crystal structure of bile acid 7α-dehydratase.Citation81
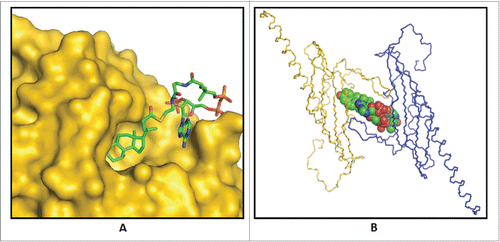
Figure 6. Predicted enzyme substrate interactions in the active site of bile acid 7α-dehydratase from Clostridium scindens. (A) Probable binding mode of 3-oxo-Δ4-chenodeoxylcholyl-CoA (3-oxo-Δ4-CDCA-CoA). Blue dashed lines and adjacent numbers are predicted interaction of His83-Nϵ2 atom with C7-OH and C6 atoms and Y30-OH group with C3-oxo atom of 3-oxo-Δ4-CDCA-CoA. The 6α-H closest to H83-Nϵ2 atom colored magenta and 6β-H away from H83-Nϵ2 atom colored brown. (B) Predicted stacking interaction involving the adenine group of the Coenzyme (CoA) moiety of oxo-Δ4-chenodeoxycholyl CoA (3-oxo-Δ4-CDC-CoA) with Y115. Carbon atoms of protein residues and product molecule are colored gold and green, respectively. H, O, N, P and S atoms are colored gray, red, blue, orange and olive, respectively.Citation81
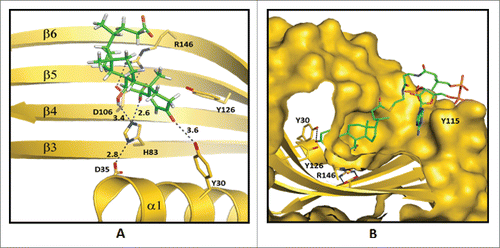
Figure 7. Proposed catalytic mechanism of bile acid 7α-dehydratase from Clostridium scindens. The role of catalytically important amino acid residues in elimination of the 7α-hydroxy group through release of a water molecule are illustrated. Tyr 30 acts as a general acid (assisted by Tyr 126) to withdraw electrons from the 3-oxo group. This allows an electron shift and destabilization of the bond holding the 6α-hydrogen. In this model, H83 accepts the 6α-hydrogen and may either protonate the 7α-hydroxy group or activate D106 or both.
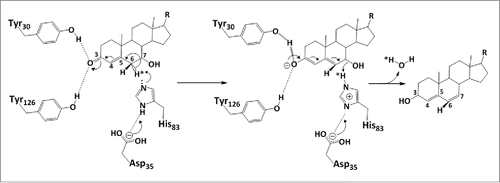
Table 1. Secondary bile acids differentially activate nuclear Receptors and G-protein coupled receptors as compared to primary bile acids.