Figures & data
Figure 1. Enterohepatic circulation and bacterial metabolism of bile acids. Primary bile acids, CA and CDCA, are synthesized in the liver from cholesterol.Citation19 In rodents, CDCA is converted to α-MCA, β- MCA Citation26 and UDCA,Citation27 the latter being considered a secondary bile acid in humans.Citation28,Citation29 Mouse-specific bile acids are represented in bold, and human-specific bile acids are represented in italics. Bile acids are conjugated with glycine or taurine (mainly taurine in rodents) before being mixed to the bile, stored in the gallbladder, and secreted to the gut.Citation19 In the gut, the microbiota deconjugates primary bile acids to release taurine and glycine.Citation30 Conjugated and deconjugated bile acids can be further dehydroxylated,Citation26 oxidated/epimerized,Citation31 esterified,Citation32 sulfated Citation33 and reconjugated Citation34 by the microbiota to produce secondary bile acids. Conversely, bile acids shape the gut microbiota composition, both directly via their detergent properties and the ability of the microbiota to metabolize bile acids, and indirectly by modulating hosts antimicrobial peptides production and immune responses.Citation2,Citation20 Primary bile acids are absorbed by the enterocytes via the ASBT transporter,Citation32 transported through the cells by IBABP, and secreted into the blood by the heterodimer OSTα/β.Citation35 Bile acids are transported into hepatocytes by NTCP and OATPs, where they activate FXR, which dimerizes with RXR to induce the transcription of SHP to inhibit CYP7A1 transcription.Citation36 In the gut, bile acids activate FXR/RXR heterodimer to induce the transcription of FGF19/15 that is secreted into the blood to reach the liver. In the liver, FGF15/19 binds to FGFR4/β-Klotho to inhibit CYP7A1 expression via FRS2α, Shp2 and ERK1/2, thus limiting de novo bile acid synthesis.Citation36 Abbreviations: ASBT, apical bile salt transporter; CYP7A1, cholesterol 7α-hydroxylase; ERK1/2, extracellular signal-regulated kinases 1/2; FGF19/15, fibroblast growth factor 19/15; FGFR4/β-Klotho, FGF receptor 4/beta-Klotho; FRS2α, fibroblast growth factor receptor substrate 2α; FXR, farnesoid X receptor; HNF4α, hepatocyte nuclear factor 4α; IBABP, ileal bile acid-binding protein; LRH-1, liver receptor homolog-1; NTCP, sodium taurocholate co-transporting polypeptide; OATPs, organic anion-transporting polypeptides; OSTα/β, organic solute transporter alpha/beta; RXR, retinoid X receptor; SHP, small heterodimer partner; Shp2, Src homology-2 domain-containing protein tyrosine phosphatase-2. Created with BioRender.com.
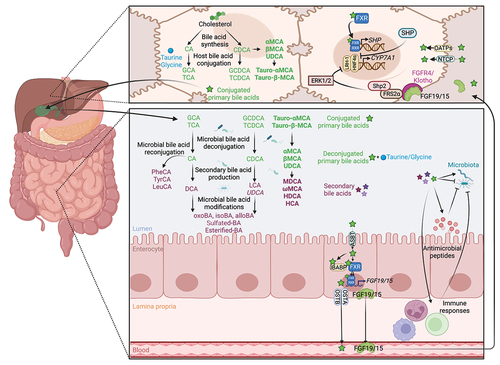
Figure 2. Structure, diversity and metabolism of known human and murine bile acids. Structure and site hydroxylation of the sterol chore for bile acids found in humans and rodents. Hydroxyl groups that are in the α-orientation are located below and are axial to the plane of the sterol chore, while hydroxyl groups in the β-orientation are located above and are equatorial to the plane of the sterol chore. Standard bile acids have the first ring in the β-trans-orientation, yielding 5β-bile acids, while allo-bile acids have this ring in the cis-orientation, yielding 5α-bile acids.Citation37 Mouse-specific bile acids are represented in bold, and human-specific bile acids are represented in italics. Created with ACD/ChemSketch, version 2021.1.2, Advanced Chemistry Development, Inc., (ADC/Labs), Toronto, ON, Canada, www.acdlabs.com.
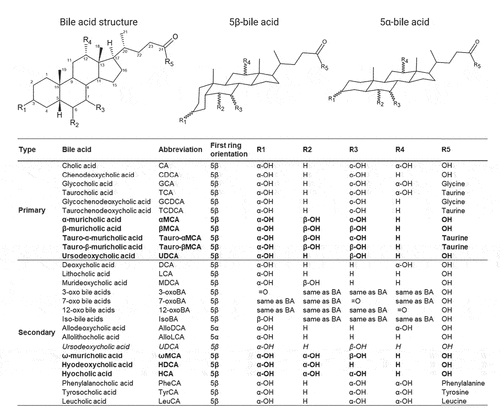
Figure 3. Bile acid-mediated regulation of intestinal barrier function. Bile acids favor the maintenance of epithelial integrity. Indeed, bile acid-mediated activation of TGR5 and FXR receptors in intestinal epithelial cells increases the expression of tight junction proteins, thus improving epithelial barrier integrity and limiting bacterial translocation and inflammation.Citation60, Citation72–74 LCA and UDCA prevents intestinal epithelial cells apoptosis and limit inflammation.Citation75 LCA-mediated activation of PXR promotes intestinal epithelial cell motility,Citation76 while TCA and DCA modulates Src/EGFR/ERK pathway activation to regulate intestinal stem/epithelial cell proliferation.Citation77 DCA also indirectly modulates intestinal stem cells proliferation and differentiation by modulating IL-22-producing type 3 innate lymphoid cells.Citation78 Bile acids also promote defense against bacterial pathogens by preventing loss of goblet cells in an FXR-dependent manner Citation73 and favoring Muc2 expression and mucin production in an FXR-independent manner.Citation79 In Paneth cells, primary bile acid-mediated TGR5 activation induces an endoplasmic reticulum stress that limits α-defensins production,Citation80 while the secondary bile acid CDCA increases the release of α-defensins by Paneth cells and of Reg3α and Reg3γ by IECs.Citation79 However, DCA inhibits FXR in Paneth cells, thus impairing their function.Citation81 Abbreviations: CDCA, chenodeoxycholic acid; DCA, deoxycholic acid; ER, endoplasmic reticulum; FXR, farnesoid X receptor; IL-22, interleukin 22; ILC3, type 3 innate lymphoid cells; LCA, lithocholic acid; PXR, pregnane X receptor; Src/EGFR/ERK Src-mediated epidermal growth factor receptor and extracellular signal-regulated kinases activation; TCA, taurocholic acid; TGR5, Takeda G protein-coupled receptor 5; UDCA, ursodeoxycholic acid. Created with BioRender.com.
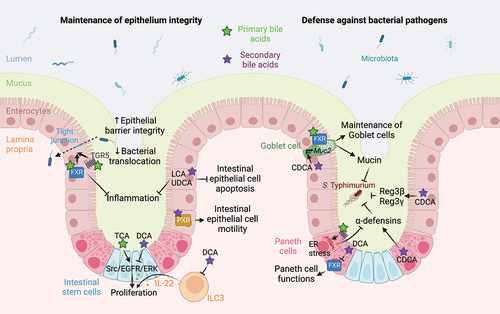
Figure 4. Microbiome-derived bile acids modulates intestinal innate and adaptive immunity. TGR5 stimulation with bile acids promotes the differentiation of monocytes into tolerogenic dendritic cells.Citation86 Bile acids promote the polarization of M0 macrophages toward an anti-inflammatory M2 phenotype by stimulating nuclear and membrane receptors.Citation87 Indeed, FXR inhibits NF-κB both indirectly by increasing SHP expression Citation88 and directly by binding to the NCor1 complex,Citation89 while PXR and VDR receptors directly repress NF-κB-mediated pro-inflammatory gene expression.Citation90,Citation91 However, TLR4 activation promotes the release of NCor1 and the activation of NF-κB-mediated gene transcription. FXR, SHP Citation92,Citation93 and the TGR5-cAMP-PKA signaling pathway Citation94,Citation95 repress inflammasome assembly and activation, thus limiting IL-1B and IL-18 production. Finally, TGR5-cAMP-PKA signaling pathway activates CREB phosphorylation, nuclear translocation, and IL-10 production Citation87,Citation96 and inhibits NF-κB-mediated pro-inflammatory gene transcription.Citation96 In CD4+ T cells, VDR activation inhibits TH1 differentiation.Citation97 Secondary bile acid epimers promote the differentiation of Treg by blocking FXR in dendritic cells,Citation98 and by activating VDR and promoting the formation of ROS in naïve CD4+ T cells.Citation99 Conversely, bile acid epimers act as an inverse agonist of RORγt in TH17 to limit their differentiation. Citation42,Citation100,Citation101 TH1 and TH17 cell exposure to bile acids drives oxidative stress, which is modulated by MDR1.Citation102 Moreover, bile acids and SCFA regulates MDR1 expression to suppress neutrophil transmigration.Citation103 Finally, B cells produce mucosal antibodies shaping the gut microbiota.Citation104 Abbreviations: cAMP, Cyclic adenosine monophosphate; CREB, cAMP response element-binding protein; FXR, farnesoid X receptor; IL, interleukin; MDR1, Multidrug Resistance Protein 1; NF-κB, nuclear factor-kappa B; NLRP3, Nod-like receptor family pyrin domain containing 3; PKA, protein kinase A; PXR, pregnane X receptor; RORγt, retinoic acid-related orphan receptor gamma t; ROS, reactive oxygen species; SCFA, short-chain fatty acids; SHP, small heterodimer partner; TGR5, Takeda G protein-coupled receptor 5; TH, T helper cell; TLR4, Toll-like receptor 4; Treg, regulatory T cell; VDR, vitamin D receptor. Created with BioRender.com.
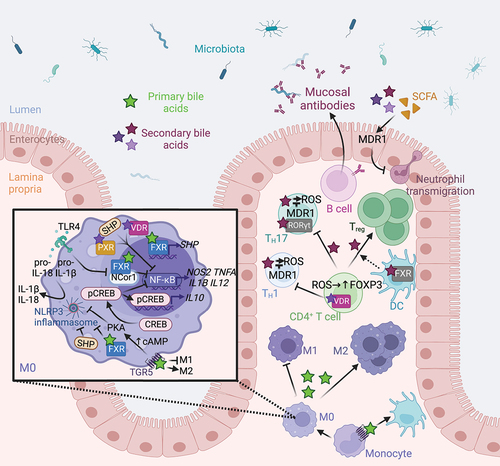
Figure 5. Microbiota-mediated bile acid metabolism and defense against pathogens. (A) Conversion of primary to secondary bile acid by the microbiota confers a colonization resistance against various pathogens. Secondary bile acids exert a direct antifungal activity against C. albicans.Citation117 The gut members C. scindens and C. sordellii produce secondary bile acids and antibiotics that act together to inhibit C. difficile growth. However, C. difficile induces secretion of primary bile acid in the gut to favor its spore germination.Citation118–120 (B) Intestinal bacteria and pathogens resist to bile acids by changing the structure of their membrane components (Omp, Lipid A, peptidoglycan), using bile acids as a source of nutrients, actively eliminating bile acids, repairing DNA damage, promoting stress responses, and forming biofilms.Citation121 (C) Bile acids promote the expression of AIEC virulence genes Citation122–124 and the use of ethanolamine and propanediol as a nitrogen and a carbon source respectively.Citation125,Citation126 Propionate generated from propanediol synergize with LPS to trigger IL-1β production and TH17 cell activation, thus promoting intestinal inflammation.Citation127 (D) Luminal bile acids increase S. Typhimurium T6SS activity and represses its T3SS until the bacteria can reach the epithelium.Citation128,Citation129 This confers a competitive advantage to the pathogen in a bile acid-rich lumen.Citation128,Citation129 Infection with S. Typhimurium decreases the ASBT-FXR-FGF15 pathway and increases inflammation and epithelial disruption.Citation23,Citation24 (E) Finally, primary bacterial infection increases the abundance of primary bile acids in the gut. Deltaproteobacteria metabolize bile acid-derived taurine to hydrogen sulfide that block pathogen aerobic respiration, thus conferring a resistance to subsequent bacterial infection.Citation25 Abbreviations: AIEC, Adherent-invasive E. coli; ASBT, apical bile salt transporter; FGF15, fibroblast growth factor 15; FXR, farnesoid X receptor; H2S, hydrogen sulfide; IL, interleukin; LPS, lipopolysaccharide; NLRP3, Nod-like receptor family pyrin domain containing 3; Omp, outer membrane porins; T3SS, type III protein secretion system; T6SS, type VI protein secretion system; TH, T helper cell. Created with BioRender.com.
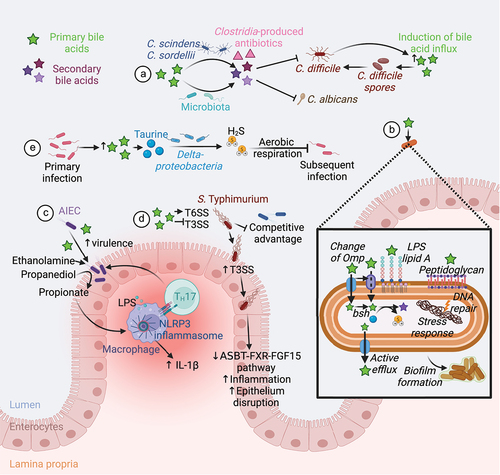