Figures & data
Figure 1. Disease-specific bacterial composition in the gut of murine model.
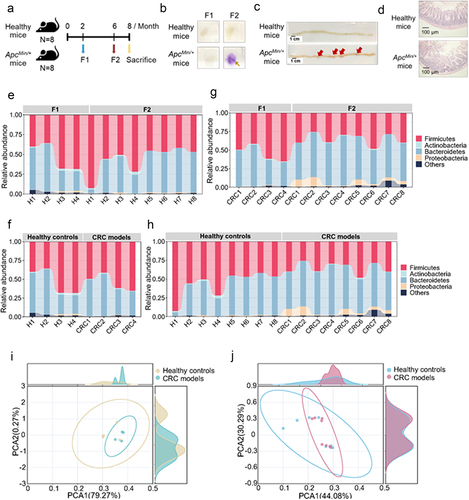
Figure 2. Potential bacterial markers of colorectal cancer.
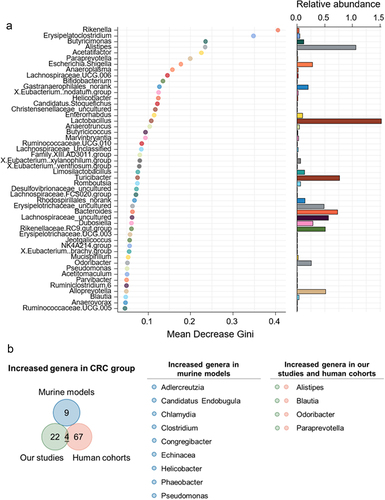
Figure 3. Relationship between gut bacteria-derived metabolites and colorectal cancer.
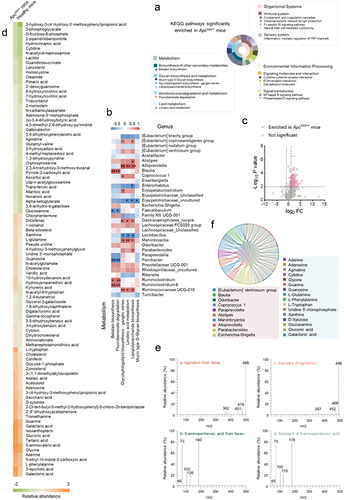
Figure 4. Promotion of inflammation by the gut metabolites to induce colorectal carcinogenesis.
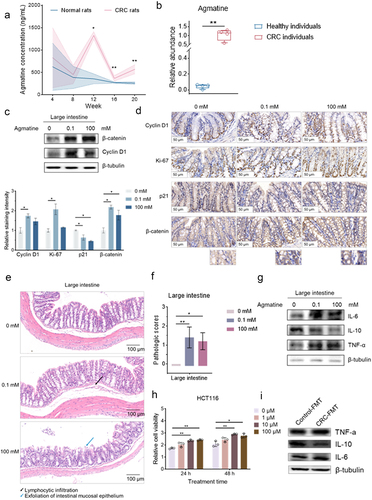
Figure 5. Underlying mechanism of agmatine-driven intestinal inflammation and adenomas in mice.
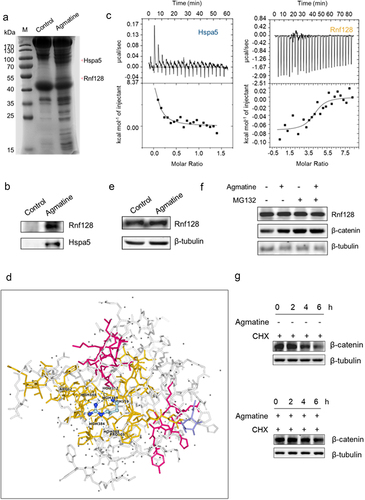
Figure 6. Promotive effects of agmatine on carcinogen-induced intestinal tumorigenesis of mice.
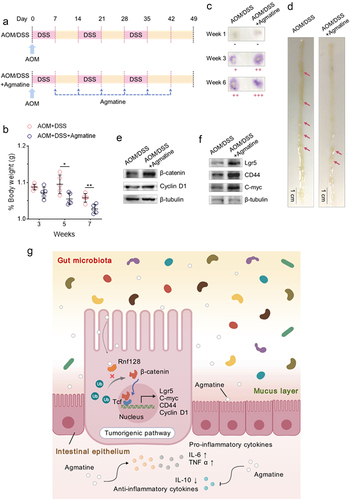