Figures & data
Figure 1. Gut microbiota regulates BA metabolism in the hepatic-intestinal circulation. BAs are synthesized from cholesterol catalyzed by CYPs in two ways in the liver, then conjugated with glycine or taurine catalyzed by BACS and BAAT. Conjugated PBAs then undergo a series of reactions including deconjugation, 7α/7β-dehydroxylation, oxidation and epimerization in the colon. Approximately 95% of the BAs reaching the terminal ileum are reabsorbed and thus recycled by the liver.
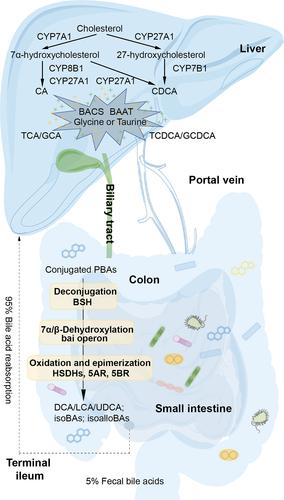
Figure 2. The deconjugation reaction and the structural features of BSH. (a) Hydrolysis of conjugated BAs by BSH to unconjugated BAs and glycine or taurine. (b) Structural homology between subunits of BSHs from Bacteroides thetaiotaomicron (PDB ID: 6UFY, blue),Citation28 Ligilactobacillus salivarius (PDB ID: 5HKE, yellow),Citation29 Bifidobacterium longum (PDB ID: 2HF0, green),Citation30 Clostridium perfringens (PDB ID: 2BJF, violet),Citation31 and Enterococcus faecalis (PDB ID: 4WL3, pink).Citation32 (c) Overall structural features of BSH, taurine and deoxycholate complex from C. perfringens (PDB ID: 2BJF). The reaction products taurine and deoxycholate are shown in pink. (d) The interactions between BSH and the substrate taurine, deoxycholate, mapped by Discovery Studio Visualizer software. (e) The catalytic mechanism of BSH.
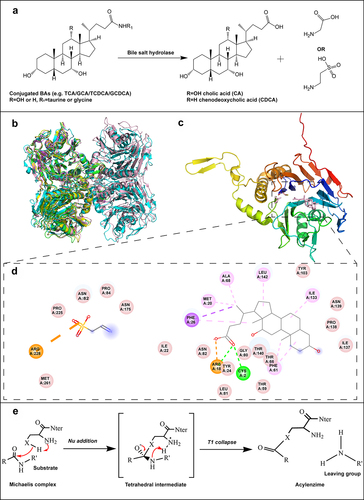
Table 1. Summary of BA biotransformation by microorganisms.
Figure 3. The dehydroxylation pathway for CA and the structural feature of 7α dehydratases. (a) Pathway of intermediate steps in 7α-dehydroxylation of CA by enzymes coded by bai operon genes. (b) The structure homology between BA 7α-dehydratases from Clostridium scindens (PDB ID: 4LEH, light pink),Citation78 Clostridium hylemonae (PDB ID: 4L8O, green),Citation79 Clostridium hiranonis (PDB: 4N3V, violet),Citation80 and Peptacetobacter hiranonis (formerly Clostridium hiranonis, PDB ID: 4L8P, blue).Citation81 (c) Predicted binding mode of 3-oxo-△4-chenodeoxylcholyl-CoA with BA 7α-dehydratases. Blue dashed lines predicted interactions of His83 and Tyr30 with substrate 3-oxo-△4-chenodeoxylcholyl-CoA.Citation82 Adapted with permission from ref. 55, copyright 2016, John Wiley and Sons. (d) Predicted stacking interaction between 3-oxo-△4-chenodeoxycholyl CoA and Tyr115. Carbon atoms of protein residues and product molecules are colored gold and green, respectively. H, O, N, P, and S atoms are colored in gray, red, blue, orange, and olive, respectively.Citation82 Adapted with permission from ref. 55, copyright 2016, John Wiley and Sons. (E) Catalytic mechanism of BA 7α-dehydratases.Citation82 Adapted with permission from ref. 55, copyright 2016, John Wiley and Sons.
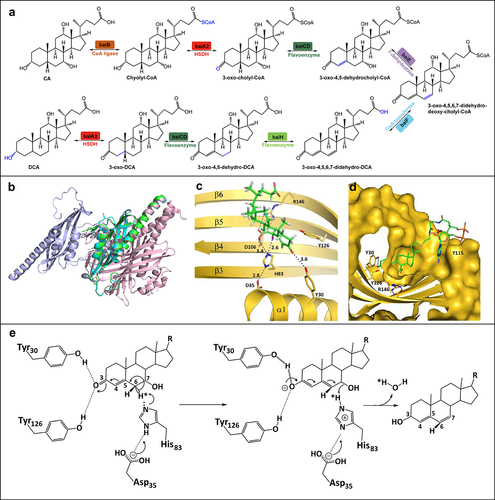
Figure 4. The oxidation and epimerization pathways of CA and CDCA and the structure features of related enzymes. (a) The pathways of CA and CDCA oxidation and epimerization. (b) Three-dimensional structures of different oxidoreductases: 3α-HSDH (PDB ID: 1FJH), 7β-HSDH (PDB ID: 5GT9), 5AR (PDB ID: 7C83), 5BR (PDB ID: 3CAQ). (c) Overall structural features of 7α-HSDH, TCDCA, and NADP+ complex from Clostridium absonum (PDB ID: 5EPO). TCDCA and NADP+ are shown in pink. (d) The interactions between CA 7α-HSDH and TCDCA, NADP+, mapped by Discovery Studio Visualizer software. (e) The catalytic mechanism of HSDHs.
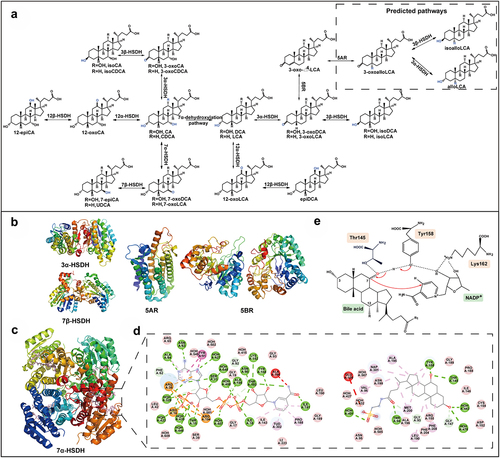
Figure 5. Interaction of gut microbiota, microbial bile acids, and host immunity. (a) Interactions between the gut microbiota and the host immune response in healthy and IBD states. Gut microbes play an important role in the maintenance of intestinal mucosal immune homeostasis, including the maintenance of an intact intestinal mucosal barrier and a modest immune response to antigens. Disturbances in the abundance and diversity of the gut microbiota can lead to impaired functioning of the host’s intestinal immune system, which can lead to IBD. (b) Bile acids can activate these receptors including LCA, 3-oxoLCA, isoLCA, isoalloLCA, DCA, isoDCA, UDCA, and OCA. IsoDCA inhibits FXR activity in DCs, resulting in the expansion of peripheral Treg cells, while OCA activates FXR to decrease the secretion of TNF-α, IL-1β, IL-6, CCL2 and to increase the level of IL-10. DCA and LCA activate TGR5 on macrophage to block NLRP3 inflammatory vesicle activity, thereby suppressing intestinal inflammation. The activation of TGR5 reduces the level of TNF-α, IFN-γ, IL-1β, IL-6, and CCL2. IsoalloLCA binds to the nuclear hormone receptor NR4A1 at the Foxp3 locus and increases Treg cell differentiation. The combination of LCA/3-oxoLCA increased the RORγt Treg cell population and proliferation via VDR. 3-oxoLCA blocks TH17 differentiation through RORγt and reduces IL-17A and IL-22 significantly. Besides, 3-oxoLCA and isoalloLCA regulate TH17 and Treg cell homeostasis. LCA also inhibits TH1 activation and differentiation via VDR.
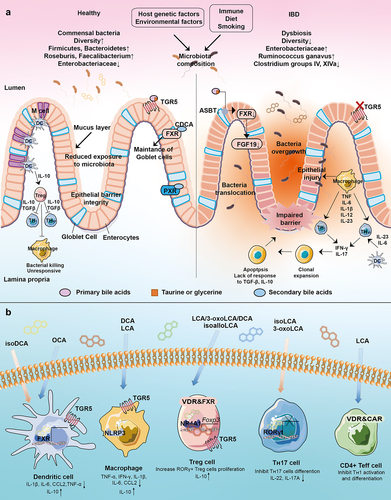
Table 2. Gut microbes and BAs changes in IBD.