Figures & data
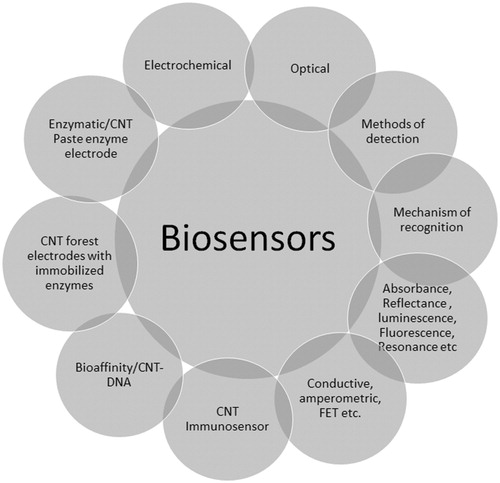
Figure 2. (a) Publications related to ‘carbon nanotubes in biosensors’ searched using ‘Web of Science’ this data includes regular articles, review articles, book chapters and conference proceedings and (b) Top 20 countries of research community working on CNTs-based biosensors.
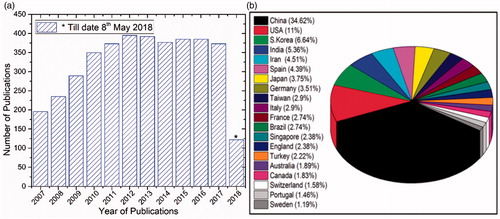
Figure 3. A schematic representation of a biosensor, the physical or chemical reaction is transformed into chemical signals after the target molecule was detected166. Where Analyte is a interest that needs to be detected (e.g. glucose is an ‘analyte’ in a biosensor designed to detect glucose); Bioreceptor is a that specifically recognizes the analyte (e.g. Enzymes, cells, aptamers, DNA and antibodies); The process of signal generation (in the form of light, heat, pH, charge or mass change, etc.) upon interaction of the bioreceptor with the analyte is termed bio-recognition; Transducer is an element that converts one form of energy into another; Electronics is the part of a biosensor that processes the transduced signal and prepares it for display; Display: The display consists of a user interpretation system such as the liquid crystal display of a computer or a direct printer that generates numbers or curves understandable by the user. This part often consists of a combination of hardware and software that generates results of the biosensor in a user-friendly manner.
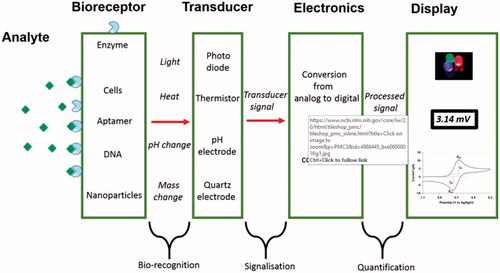
Figure 4. Different surface modifications of CNTs [Citation185]. (a) Aromatic molecules can be appended to nanotubes using certain non-covalent interactions (known as π–π interactions). Groups emanating from these molecules interact with the surrounding solvent or matrix. (b) Non-covalent interactions (including π–π interactions, van der Waals forces and charge-transfer interactions) can be used to wrap polymers around nanotubes and (c) Chemical groups can be covalently attached to nanotubes. In this case, lithium (Li) forms anions on the nanotube, which react with styrene monomers to form polystyrene chains covalently attached to the nanotube walls.
![Figure 4. Different surface modifications of CNTs [Citation185]. (a) Aromatic molecules can be appended to nanotubes using certain non-covalent interactions (known as π–π interactions). Groups emanating from these molecules interact with the surrounding solvent or matrix. (b) Non-covalent interactions (including π–π interactions, van der Waals forces and charge-transfer interactions) can be used to wrap polymers around nanotubes and (c) Chemical groups can be covalently attached to nanotubes. In this case, lithium (Li) forms anions on the nanotube, which react with styrene monomers to form polystyrene chains covalently attached to the nanotube walls.](/cms/asset/ae350bdf-a302-44d3-a08a-075fc4878f27/ynan_a_1478765_f0004_c.jpg)
Figure 5. Covalent and non-covalent interactions between CNTs and linear, dendritic and hyperbranched polymers and also biomacromolecules for producing CNT–polymer hybrid nanomaterials as anticancer drug delivery systems and Surface functionalization of the CNTs for biomedical applications [Citation186].
![Figure 5. Covalent and non-covalent interactions between CNTs and linear, dendritic and hyperbranched polymers and also biomacromolecules for producing CNT–polymer hybrid nanomaterials as anticancer drug delivery systems and Surface functionalization of the CNTs for biomedical applications [Citation186].](/cms/asset/5d072866-9627-450e-a8d3-265c3cb04910/ynan_a_1478765_f0005_c.jpg)
Figure 6. Surface chemistry used to immobilize proteins on gold-coated glass slides for Raman detection of analytes by SWNT Raman tags. A self-assembled monolayer of cysteamine on gold was covalently linked to six-arm, branched poly (ethylene glycol)-carboxylate (6arm-PEG-COOH, right) to minimize nonspecific protein binding. Terminal carboxylate groups immobilize proteins. (b) Sandwich assay scheme. Immobilized proteins in a surface spot were used to capture an analyte (antibody) from a serum sample. Detection of the analyte by Raman scattering measurement was carried out after incubation of SWNTs conjugated to goat anti-mouse antibody (GaM-IgG-SWNTs), specific to the captured analyte. SWNTs were functionalized by (DSPE-3PEO) and (DSPE-PEG5000-NH2) (left). (c) Raman spectra of the SWNT G mode and radial breathing mode (RBM, inset) regions before and after SERS enhancement [Citation101].
![Figure 6. Surface chemistry used to immobilize proteins on gold-coated glass slides for Raman detection of analytes by SWNT Raman tags. A self-assembled monolayer of cysteamine on gold was covalently linked to six-arm, branched poly (ethylene glycol)-carboxylate (6arm-PEG-COOH, right) to minimize nonspecific protein binding. Terminal carboxylate groups immobilize proteins. (b) Sandwich assay scheme. Immobilized proteins in a surface spot were used to capture an analyte (antibody) from a serum sample. Detection of the analyte by Raman scattering measurement was carried out after incubation of SWNTs conjugated to goat anti-mouse antibody (GaM-IgG-SWNTs), specific to the captured analyte. SWNTs were functionalized by (DSPE-3PEO) and (DSPE-PEG5000-NH2) (left). (c) Raman spectra of the SWNT G mode and radial breathing mode (RBM, inset) regions before and after SERS enhancement [Citation101].](/cms/asset/5196d777-e712-4c4f-b122-9781d5a1dfed/ynan_a_1478765_f0006_c.jpg)
Table 1. Recent developments in biosensors based on SWCNTs/MWCNTs.
Figure 7. Schematic representation of the SWCNT immunosensor and its selective CD4 T cell capture [Citation26].
![Figure 7. Schematic representation of the SWCNT immunosensor and its selective CD4 T cell capture [Citation26].](/cms/asset/7c60e587-8598-430b-92cc-644fbe933f00/ynan_a_1478765_f0007_c.jpg)
Figure 8. Electrochemical and electronic CNT biosensors for cancer detection. (A) Schematic illustration of the folic acid-targeted cytosensing strategy for an enhanced electrochemical detection of cancer cells using polydopamine-coated CNTs. (B) Schematic representation of an electrochemical DNA biosensor for cancer detection based on gold nanoparticles/aligned CNTs [Citation187].
![Figure 8. Electrochemical and electronic CNT biosensors for cancer detection. (A) Schematic illustration of the folic acid-targeted cytosensing strategy for an enhanced electrochemical detection of cancer cells using polydopamine-coated CNTs. (B) Schematic representation of an electrochemical DNA biosensor for cancer detection based on gold nanoparticles/aligned CNTs [Citation187].](/cms/asset/c21c7522-e073-4597-bb41-93ea3e9f70e4/ynan_a_1478765_f0008_c.jpg)
Figure 9. SWCNT/luciferase hybrid for cellular ATP detection: (a) SWCNT-based sensor that is able to selectively recognize ATP against other interfering molecules such as AMP, AMP, CTP and GTP, which allows to spatiotemporally detect ATP in living cells. The oxidized production of d-luciferin, oxyluciferin, quenches the NIR fluorescence of SWCNT. (b) NIR fluorescence images of HeLa cells containing SWCNTLuc sensor (2 μg/mL) with and without addition of Lrin. The NIR fluorescence in HeLa cells is quenched after addition of Lrin (240 μM), which is correlated with the ATP concentration. Reproduced with permission from [Citation131].
![Figure 9. SWCNT/luciferase hybrid for cellular ATP detection: (a) SWCNT-based sensor that is able to selectively recognize ATP against other interfering molecules such as AMP, AMP, CTP and GTP, which allows to spatiotemporally detect ATP in living cells. The oxidized production of d-luciferin, oxyluciferin, quenches the NIR fluorescence of SWCNT. (b) NIR fluorescence images of HeLa cells containing SWCNTLuc sensor (2 μg/mL) with and without addition of Lrin. The NIR fluorescence in HeLa cells is quenched after addition of Lrin (240 μM), which is correlated with the ATP concentration. Reproduced with permission from [Citation131].](/cms/asset/07219313-254a-4ffe-9816-aaf05d0e78fc/ynan_a_1478765_f0009_c.jpg)
Figure 10. (top) Scanning electron micrograph of the array of CNTFETs. S: source. D: drain. G: contact to back gate. 896 pairs of S/D electrodes are packed in 1 cm2. (bottom) Schematic.
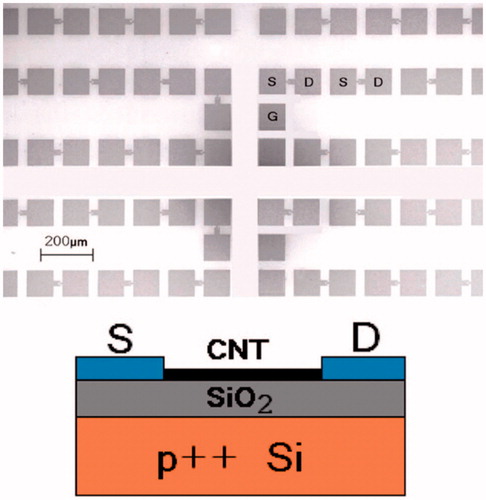