Figures & data
Figure 1. Validation of in-vitro-generated mΦ. (A) CD14+ MACS-isolated peripheral blood monocytes from healthy donors were cultured with HPNE-, Panc1- and MiaPaCa2-conditioned media for 7–9 d. The representative bright-field images of mΦ generated from the respective conditioned media are shown. (B) The three mΦ types were assayed for their ability to endocytose using pHrodo-Red Dextran beads. The histograms indicating uptake of the pH-sensitive red fluorescence after 2 and 20 min incubation with the beads are shown. (C) The histograms of the expression of classical mΦ markers CD68, CD163, M-CSFR and CD206 on the three mΦ types by flow cytometry are shown. Dotted lines through CD163 and CD206 histograms have been drawn to demarcate peak of HPNE mΦ stain. (A–C) First (green), second (red) and third (blue) rows denote data for HPNE, Panc1 and MiaPaCa2 mΦ, respectively. Data are representative of n = 4 independent experiments. (D) The histograms of Annexin-V staining by flow cytometry on the mΦ types are shown. Bar graphs depict the mean ± SEM of the proportion of Annexin-V+ apoptotic cells and DAPI− live cells of total cells for the three mΦ types. Data are collated from n = 2 independent experiments. (E) MΦ are assayed for transcript expression of M1 and M2 markers IL-1β, IL-6, TNF-α, IL-10 and Arg1 by qPCR. Bar graphs shown are the mean ± SEM of the relative expression of that gene over HPRT. Data are representative of n = 3 independent experiments. *p < 0.05; **p < 0.01; ***p < 0.001.
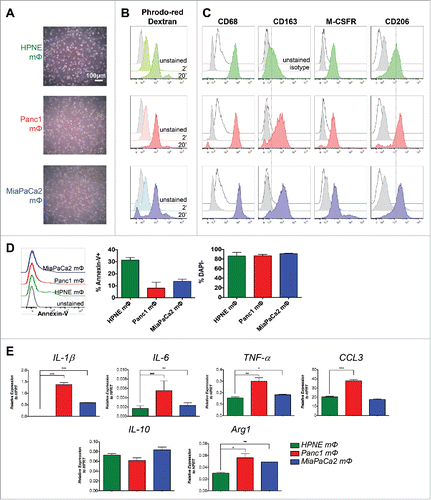
Figure 2. Panc1 and MiaPaCa2 mΦ promote higher rates of angiogenesis compared to HPNE mΦ. MΦ supernatants (sups) harvested from the three mΦ types were added to 1 × 104 HUVECs grown on top of matrigel per well of Ibidi's μ-angiogenesis slide for 4 h at 37°C. 200 ng/mL rhVEGF-A and 10 μM of VEGFR inhibitor Sorafenib were used as a positive and negative control, respectively. (A) The representative bright-field images of HUVEC networks analyzed by Ibidi's WimTube Image Analysis software are shown. (B) Total network length (networks identified by red lines, length of tubes identified by blue regions), (C) total branch points, (D) total loops (identified by small yellow numbers) and (E) total nets are depicted in bar graphs as mean ± SEM of collated data from n = 6 independent experiments. (F) A bar graph of mean ± SEM of the expression of VEGFA by qPCR in the respective mΦ is shown. Data are representative of n = 3 independent experiments. (G) Shown are bar graphs of total network length in which VEGFA is neutralized in the macrophage supernatants by the addition of an anti-VEGF antibody. Data is collated from n = 4 independent experiments. *p < 0.05; **p < 0.01; ***p < 0.001.
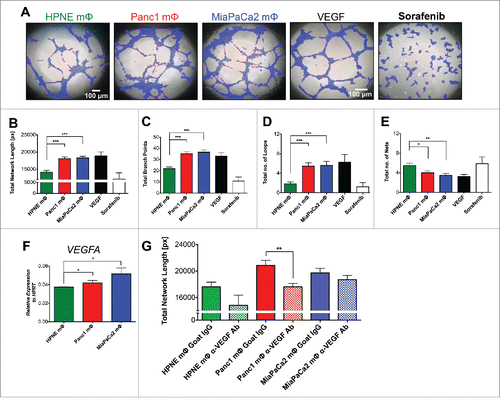
Figure 3. Panc1 and MiaPaCa2 mΦ augmented extravasation compared to HPNE mΦ. A PDMS microfluidic device consisting of a central gel region flanked by two side fluidic channels was utilized. HUVECs were cultured in a uniform monolayer mimicking a blood vessel wall in one of the fluidic channels. Panc1 or MiaPaCa2 tumor cells with or without corresponding mΦ (1:1) were co-injected into the channel containing HUVECs. After 24 h, tumor cell extravasation across the endothelial monolayer into the gel region was quantified by confocal microscopy and expressed as a percentage of the total number of cells present on the monolayer. (A) 3D drawing of the microfluidic device and an illustration of the expected movement of tumor cells and/or mΦ across the HUVEC monolayer into the 3D matrix are shown. This mimics the extravasation of cells from blood vessels into potential secondary metastatic sites. (B) The representative immunofluorescence images of MiaPaCa2 tumor cells in three different conditions after 24 h are shown: (i) not extravasated, (ii) in the process of extravasating and (iii) extravasated across the HUVEC monolayer. (C) Shown are bar graphs of mean ± SEM of the percentage of extravasated cancer cells, using collated data from at least six devices per condition. **p < 0.01;***p < 0.001.
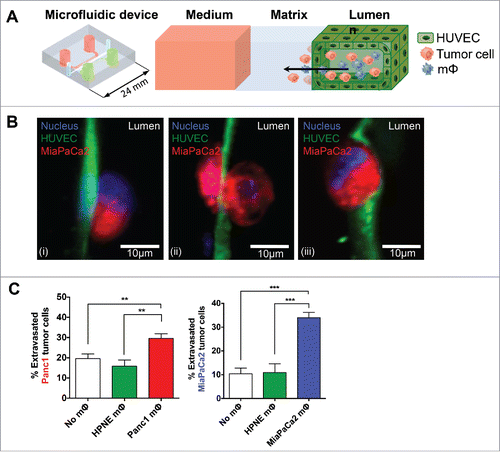
Figure 4. Panc1 and MiaPaCa2 mΦ induced higher rates of EMT compared to HPNE mΦ. 3 × 103 HPAF-II (epithelial pancreatic tumor cell line) cells in single cell suspension were seeded per well in 96-well optical bottom plates and allowed to grow into tight self-contained colonies for 4 d. On day 4, media was aspirated from wells and mΦ supernatants were added to the HPAF-II colonies to induce EMT. After 36–48 h, HPAF-II colonies were assayed by confocal microscopy to determine three key parameters : (i) colony spreading (increase in internuclear distance of neighboring cells within each colony), (ii) loss of epithelial marker (E-cadherin) expression, (iii) morphological “flattening” of cells (increase in area) (A) The representative immunofluorescence images of HPAF-II colonies incubated with the respective mΦ supernatants, stained with E-cadherin and phalloidin are shown. (B) A collated scatter plot of mean ± SEM of the internuclear distance is shown. Each dot represents a measurement between two neighboring HPAF-II cells. (C, D) The collated scatter plots of mean ± SEM of the intensity of E-cadherin (C) and area of F-actin (D) where each dot represent a single HPAF-II cell are shown. (B–D) Data for scatter plots were tabulated from TissueQuest-analyzed images of at least eight colonies per condition. Data are representative of n = 8 (A–D) independent experiments. (E) The bar graphs of mean ± SEM of the transcript expression of TGFβ and HGF of the macrophages by qPCR are shown. Data are representative of n = 3 independent experiments. (F) The collated scatter plots of the mean ± SEM of the internuclear distance from n = 4 independent experiments are shown, in which HGF was neutralized with an anti-HGF antibody (Goat IgG as isotype control), and TGFβ was neutralized with an anti-TGFβ antibody (Mouse IgG1 as isotype control) in the macrophage supernatants. *p < 0.05; **p < 0.01; ***p < 0.001; ns = not significant.
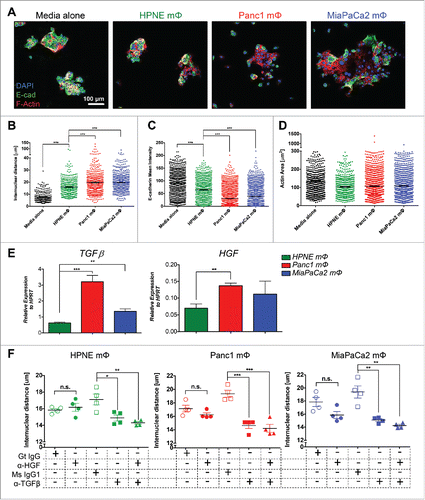
Figure 5. Panc1 and MiaPaCa2 mΦ exhibit pronounced glycolytic profile compared to HPNE mΦ. The representative line graphs of the mean ± SEM of ECAR (A) and OCR (B) values at each timepoint in the Glycolysis Stress test and Mitostress test, respectively, performed on the three mΦ types are shown. Beside the line graphs are bar graphs of the mean ± SEM of calculated glycolytic capacity (A) and maximal mitochondrial respiration capacity (B). Data are representative of n = 9 independent experiments. (C) MΦ are assayed for transcript expression of key glycolysis genes HK1, HK2, GPI, ALDOA, TPI1 and PGK1 by qPCR. Bar graphs shown are the mean ± SEM of the relative expression of that gene over HPRT. Data are representative of n = 4 independent experiments. (D) A bar graph of the mean ± SEM of the fold change of L-lactate measured by LC-MS of the Panc1 and MiaPaCa2 MΦ lysates with respect to HPNE MΦ lysates is shown. Data is collated from n = 4 independent experiments. (E) The histograms of ROS production, from mΦ induced with a known ROS inducer as a positive control (left panel) and from the three mΦ types at basal level (right panel) are shown. Bar graph below shows the mean ± SEM of ROS MFI collated from n = 2 independent experiments. *p < 0.05; **p < 0.01;***p < 0 .001.
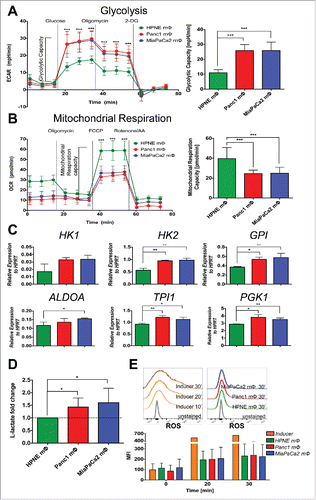
Figure 6. 2DG inhibition of glycolysis in Panc1 and MiaPaCa2 mΦ reverses pro-metastatic phenotype. After 7–9 d of monocytes co-cultured with HPNE, Panc1 and MiaPaCa2 conditioned media to obtain the three mΦ types, media was aspirated and replaced with fresh media with or without 25 mM 2DG for the next 6 h. MΦ supernatants were then harvested and used for downstream angiogenesis (A, B), extravasation (C) and EMT (D–G) assays. (A) The representative bright-field images of HUVEC networks analyzed by Ibidi's WimTube Image Analysis software are shown, along with (B) scatter plots of the mean ± SEM of the key metrics as described in . Data shown is collated from n = 6 independent experiments. (C) The bar graphs of the mean ± SEM of the percentage of extravasated cancer cells, using collated data from at least four devices per condition are shown. ***p < 0.001. (D) The representative immunofluorescence images of HPAF-II colonies incubated with the respective mΦ supernatants, stained with E-cadherin and phalloidin are shown. (E–G) The scatter plots of the mean ± SEM of internuclear distance (E), mean intensity of E-cadherin (F) and F-actin (G) and the area of F-actin (G) are shown. (E–G) Scatter plots are data of one experiment from at least eight colonies per condition. Data are representative of n = 6 independent experiments.
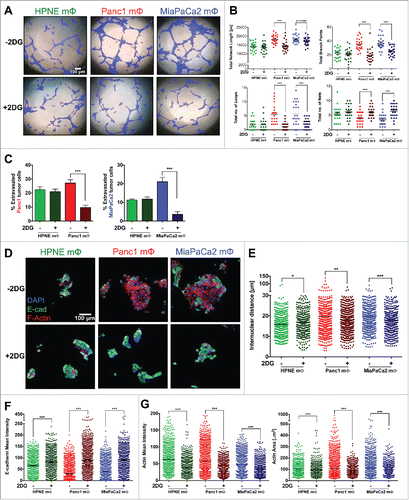
Figure 7. TAMs support metastatic dissemination and colonization at secondary sites. MΦ that infiltrate PDAC lesions adopt a metabolic profile reminiscent of the Warburg effect for which PDAC tumor cells also exhibit.Citation50 These infiltrating mΦ support tumor cell dissemination by promoting (1) angiogenesis, inducing (2) EMT and augmenting (3) extravasation of tumor cells to potential secondary sites. Sustained inhibition of such high rates of glycolysis using 2DG in TAMs subverts all three pro-metastatic capacities. Taken together, our results highlight the potential of pharmacologics targeted at modulating metabolic pathways that deliver a one–two punch to both immune cells and tumor cells, thus refining our approach to curtailing metastasis in PDAC.
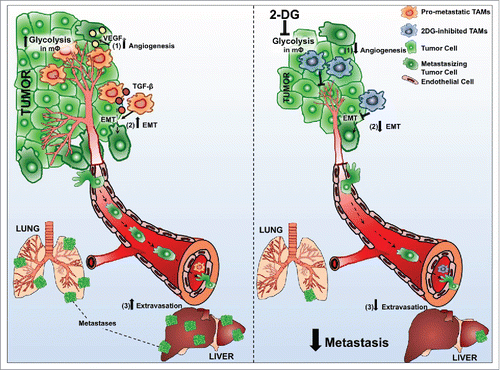