Figures & data
Figure 1. Peptide overall strategy. (i) Prediction of B-cell epitopes based on multiple computerized antigenicity/immunogenicity algorithms. This represents a critical component in the overall process; (ii) B-cell linear epitopes are refined through mutagenesis studies, and epitopes are modeled based on the 3-dimensional native antigen structure and antigen:antibody complex if available. The unique B cell epitope peptides are engineered to recapitulate the exquisite native structure of the tumor antigen. This is a crucial prerequisite to developing high affinity antibodies given that B-cell epitopes can be both linear (contiguous amino acid sequence) and conformational (discontinuous in the primary sequence but close in proximity of the folded 3D structure) play an important role; (iii) The chimeric vaccine is designed to include a “promiscuous” T cell epitope as a vital component in the development of a universal vaccine for an outbred population that can bypass haplotype restricted immune responses; (iv) Highly efficacious native-like antipeptide-antibodies are then elicited in animals when the vaccine is emulsified with an adjuvant (nor-MDP) in a squalene-arlacel oil-water ISA720 vehicle; (v) Stable peptide mimics are designed as retro-inverso analogs that can block receptor:ligand interactions; (vi) Both antibodies and peptide mimics are used in a series of in vitro assays in different human cell lines to evaluate their efficacy. By corroborating the data we are able to reduce the numbers of lead candidates to a minimum; (vii) Combinations of the best performing epitopes are assessed in combination in human cell lines to determine synergy/additivity; (viii) In vitro testing of potential combinations in different tumor cell lines using NOD/SCID mice as a surrogate animal model to simulate human cancers and evaluate the effects of peptide antibodies elicited in response to the vaccine epitopes in different tumor cell lines; (ix) the combinations are then tested using SCID mouse models as a surrogate animal model to simulate human cancers and evaluate the effects of peptide antibodies elicited in response to the vaccine epitopes to finalize the potential candidates; (x) Test efficacy in transgenics or appropriate animal model; (xi) Finally, we conduct human clinical trials to assess safety and toxicity.
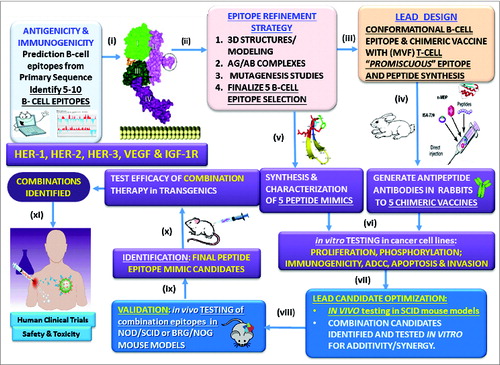
Figure 2. Roadmap of peptide vaccine versus peptide mimic strategies. Pathway A involves the design of chimeric peptides comprising the conformational B-cell epitope and incorporating a “promiscuous” T-cell epitope. The vaccine is emulsified with an adjuvant (nor-MDP) in a vehicle (ISA 720; squalene/arlacel) (C), and used for vaccination. When relevant transgenic mice (MMTV/neu) or a syngeneic model) (D1) are available for assessing vaccine efficacy, these mice are immunized sc with the chimeric peptide vaccine and tumor growths/reductions are monitored over time. Rabbits are used as a surrogate model (D2) to develop high affinity antipeptide antibodies which can be used to treat transplantable mouse models (SCID) (E1) after tumor challenge. Tumor growth is then monitored over time as we have described. The humoral response (D3) results in activated B-lymphocyte (D4) and the plasma cell give rise to high affinity antibodies (D5) that recognizes the antigen (D6) on tumor cells causing cell death through several different mechanisms (D7) (ADCC, Apoptosis, etc). On the other hand, Pathway B involves the design of conformational B cell-peptide mimics with enhanced proteolytic stability. Because a T-cell epitope is not included, the peptide lacks immunogenicity, thus the peptide mimic treatment does not elicit antibody production. The peptide mimics are dissolved in PBS and are injected in mice by iv treatment (E2) at the time of tumor challenge, and treated repeatedly over several weeks until control mice develop large tumors. This strategy allows us to corroborate effectiveness of antibody treatment vs. peptide treatment, permitting us to prioritize peptide constructs for testing in (i) transgenic mouse models (F); and (ii) human clinical trials (G). The peptide mimics bind the receptor (E3) and inhibits signal transduction (E4) resulting in tumot cell death.
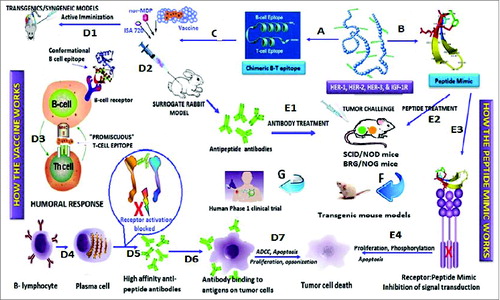
Figure 3. Schematics of retro-inverso peptides. (A) Parent peptide, synthesized with L-amino acids. (B) Parent peptide, synthesized with D-amino acids; side chains in opposite orientation. (C) Retro-inverso peptide (amino acid sequence reversed in relation to the parent peptide) synthesized with D-amino acids in reverse order (RI); Reversal of the peptide backbone direction will result in the mirroring of protein structure while inversion of amino acid chirality (L to D) achieves a mirroring of side chain positions (exact mirror image of parent peptide).
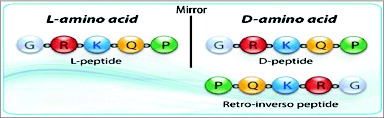
Figure 4. Signal transduction pathways drive cancer metastasis. The signaling pathways of HER family members, VEGF and IGF-1R and the current drugs that target these oncoproteins in cancer are shown. HER-2 can heterodimerize with any of the ligand-activated HER receptors (HER-1, HER-3 or HER-4) and this association leads to intracellular signaling via 2 major pathways, the MAPK pathway and the PI3K pathway, leading to proliferation, cell survival, metastasis and angiogenesis. On the other hand, VEGF can bind to its main receptor, VEGFR-2 (KDR), and this binding causes intracellular phosphorylation of the receptor, thereby stimulating the PI3K pathway and stimulating angiogenesis. The signaling pathways can be targeted extracellularly using humanized monoclonal antibodies, such as trastuzumab and pertuzumab (HER-2), cetuximab (EGF receptor), bevacizumab (Avastin) (VEGF), and cixutumumab (IGF-1R) which can prevent ligand binding and activation of the receptors or can directly block binding of an activated receptor to another. At the intracellular level, small-molecule inhibitors, such as sunitinib (VEGF), lapatinib (HER-1 and HER-2) and erlotinib (HER-2), can disrupt the phosphorylation sites and directly prevent activation of the PI3K or MAPK pathways. P: Phosphate; VEGFR: VEGF receptor.

Table 1: Expression of HER and IGF-1R in human cell lines
Table 2: HER-2 & IGF-1R
Table 3: HER-1 & IGF-1R
Table 4: HER-3 & IGF-1R
Table 5: HER-3 & HER-2