Figures & data
Figure 1. In situ vaccination enhances immunogenicity and drives effective cell-mediated anti-tumor immune responses. The activation of APCs through triggering ‘danger receptors’ like Toll-like receptors TLRs while concomitantly exposing APCs to tumor antigens leads to production of proximal immune activating cytokines, in particular, the IL-1 cytokine. Th0 cells are CD4+ cells, which are not yet committed to a distinct differentiation path and are influenced by the dominant local cytokine milieu to express distinct nuclear transcription factors, leading to differentiation into either Th1 (Tbet), Th2 (GATA-3), Th17 (RORγT) or Treg (FOXP3). Upstream production of IL-1 together with IL-12 leads to expression of IFNγ, which in turn leads to further increases in IL-12 and IFNγ production and sensitivity, driving a feed-forward loop that locks-in a Th1-associated immune response, characterized by NK cells and cytotoxic CD8+ generation and activation. Exposure of Th0 cells to cytokines like IL-4, TGFβ or IL-23 can drive the differentiation of CD4 T cells to a Th2, Treg or Th17 phenotype. Although there is limited data on whether Th17 skewing leads to effective anti-tumor immunity, the generation of Tregs and a strong Th2 bias appear to suppress effective anti-tumor responses. By driving IL-12/IFNγ production, In Situ vaccination leads to a strongly biased Type 1-associated cell-mediated immune response required for effective anti-tumor immunity. A potential benefit of intratumoral vaccination is that antigens are presented to the immune system through the induction of immunogenic tumor cell death, obviating the need to choose a priori the potentially therapeutic antigen or set of antigens for a particular patient.
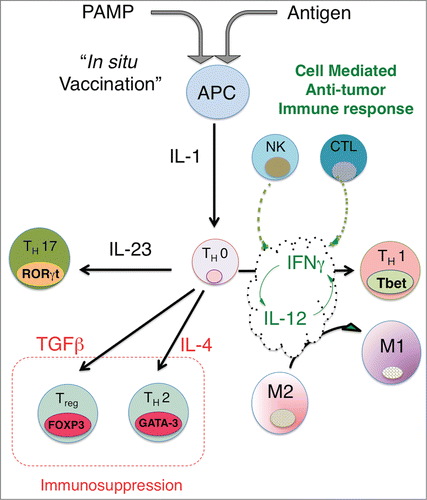
Figure 2. Close spatial association of PD-1+ and PD-L1+ cells suggests induction of ‘adaptive resistance’. Immunohistochemical (IHC) staining was performed to detect either PD-1 (A), (C, green) or PD-L1 (B), (D, red) on adjacent 5 micron sections of formalin-fixed paraffin-embedded tumor samples from a patient with HPV+ squamous cell carcinoma of tonsil. E and F are composite images of C &D. The close physical proximity of PD-1+ small mononuclear cells and larger PD-L1+ cells is ‘adaptive resistance’ – the inductive up-regulation of PD-L1 in response to the influx of tumor-reactive CD8+ T cells. Although initially described in the context of metastatic melanoma, ‘adaptive resistance’ may be a common pattern of immune subversion in many types of tumors, indicating the “activation” of the PD-L/PD-1 axis and likelihood of response to agents that block this pathway. IHC was performed with anti-PD-L1 (mouse anti-human mAb5H1 clone) followed by a secondary anti-mouse IgG (DAKO, USA) or anti-PD-1 (goat anti-human polyclonal antibody, R&D Systems) followed by a secondary biotinylated anti-goat IgG (Jackson ImmunoResearch, USA). For amplification, horse radish-peroxidase (HRP) was used and the reaction visualized with the DAB chromogen enzyme. Dual immunofluorescent IHC was performed by sequential staining with anti-PD-L1 (detected by red-fluorescent Alexa Fluor 647 tyramide, followed by anti-PD-1 detected by green-fluorescent Alexa Fluor 488 tyramide).
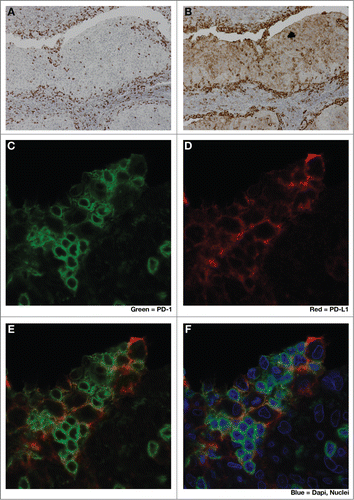
Figure 3. Induction of Tregs correlates with decreased progression-free survival in patients treated with low-grade B cell lymphoma. Fifteen patients were treated with intratumoral injection with a TLR9 agonist (PF-3512676, 6 mgs per injection) into a single tumor followed by low-dose radiation. The TLR9 agonist was injected immediately prior to radiotherapy, after a second dose of radiotherapy, then weekly for up to 8 weeks. Clinical responses were evaluated based on assessment of non-injected/non-irradiated lesions. (A) The ability of CpG-activated tumor B cells to stimulate induction of Tregs (CD25+FOXP3+CD4+) cells was assessed by incubating peripheral lymphocytes isolated from the blood of pre-vaccinated patients either with media alone or tumor cells isolated from tumor sites treated with CpG and low-dose radiation. Flow cytometric analysis revealed a dichotomous Treg-induction phenotype with one group showing minimal increases in Tregs upon co-incubation with malignant, treated B cells (“Treg-Non-Inducer”) and the other group demonstrating enhanced Treg induction (“Treg-Inducer”). (B) Progression-free survival of these two groups revealed a significant correlation of Treg induction with decreased PFS (P = 0.0058), suggesting that induction of a Treg response may limit the effectiveness of in situ vaccination therapies. Adapted from Brody-JD, JCO V28 N28, 2010.Citation13 Used by permission.
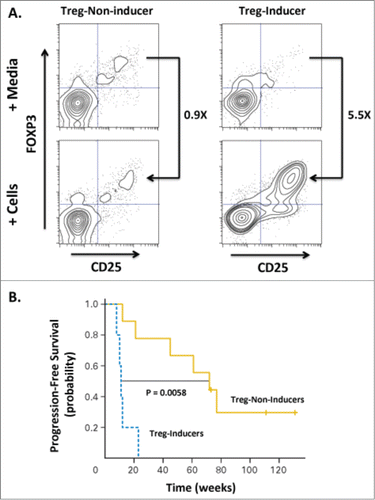