Figures & data
Figure 1. Increasing number of immunology studies involving in silico approaches over the last decade. Metrics were extracted from NCBI PubMed for search terms indicated. In the case of epitope prediction, keywords were linked together within quotations in order to return relevant results.
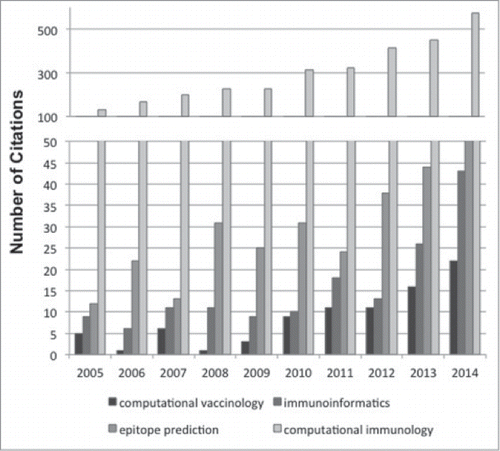
Figure 2. Computational immunology word cloud. Computational immunology Google search term results were input into the Wordle word cloud generator (http://www.wordle.net/) that sizes terms by frequency. The Google search for computational vaccinology terms was performed in March 2015.
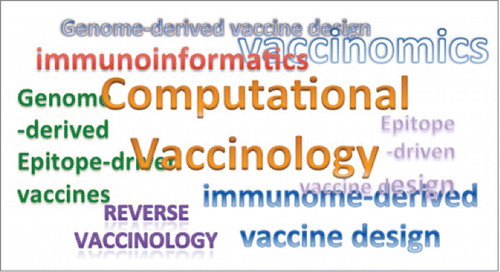
Figure 3. Integration of iVAX into the epitope-driven vaccine development pipeline. A development pathway from genome to vaccine is shown. Pathogen-derived genetic sequences obtained from various sources are initially built into a database in iVAX. T cell epitope vaccine candidates are selected using epitope identification and homology algorithms according to user-defined preferences for HLA coverage, pathogen strain coverage and T cell cross-reactivity. Vaccine construction algorithms assemble epitope ensembles into multi-epitope sequences containing the necessary and sufficient epitope content to activate strong T cell responses specific to the target pathogen. iVAX accelerates epitope identification in comparison with experimental methods using overlapping peptide libraries, for example. In silico predictions are confirmed using in vitro methods and vaccines are produced and tested for immunogenicity and efficacy.
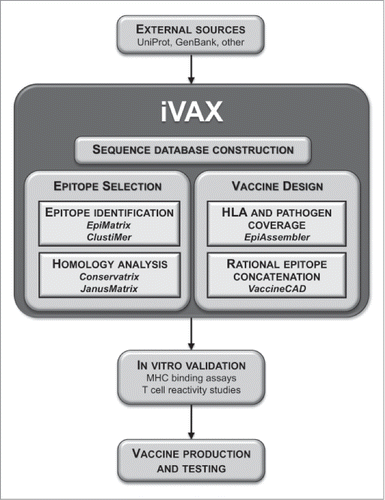
Figure 4. Immune Camouflage. Bacteria and viruses have evolved a number of subterfuges to escape immune response. A recently discovered strategy is to disguise T cell epitopes that may be presented by antigen presenting cells as “self proteins” that are recognized by Tregs (pink and gray) rather than T effector epitopes (blue).
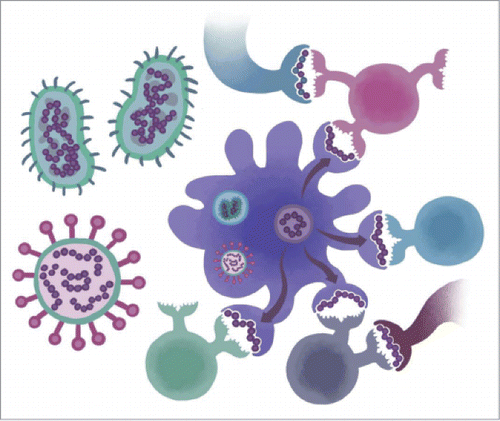
Figure 4. (B) JanusMatrix, Epitope Networks for a Regulatory and Effector T cell epitopes. T cell epitope networks were produced for a regulatory T cell epitope from hepatitis C virus (A) [24] and an effector T cell epitope from influenza (B) [47], using JanusMatrix and Cytoscape. Potentially cross-reactive T cell epitopes were identified by JanusMatrix, which searches proteins in human genome (left), human microbiome (center), and human pathogen genome (right) databases for HLA ligands with TCR-facing residues identical to each of the query epitopes. Green diamonds represent source peptides; gray squares are predicted 9-mer epitopes derived from the source peptide; blue triangles are 9-mers that are 100% identical to the TCR face of the source epitope and that are predicted to bind to the identical HLA; and light purple circles (on the outer rim of the array) are proteins containing the cross-reactive epitope.
![Figure 4. (B) JanusMatrix, Epitope Networks for a Regulatory and Effector T cell epitopes. T cell epitope networks were produced for a regulatory T cell epitope from hepatitis C virus (A) [24] and an effector T cell epitope from influenza (B) [47], using JanusMatrix and Cytoscape. Potentially cross-reactive T cell epitopes were identified by JanusMatrix, which searches proteins in human genome (left), human microbiome (center), and human pathogen genome (right) databases for HLA ligands with TCR-facing residues identical to each of the query epitopes. Green diamonds represent source peptides; gray squares are predicted 9-mer epitopes derived from the source peptide; blue triangles are 9-mers that are 100% identical to the TCR face of the source epitope and that are predicted to bind to the identical HLA; and light purple circles (on the outer rim of the array) are proteins containing the cross-reactive epitope.](/cms/asset/bb85fe98-1812-44a6-8d2e-4334d3e304b1/khvi_a_1061159_f0004b_oc.gif)
Figure 5. JanusMatrix prediction of epitope cytokine profiles. A retrospective survey of epitope-specific cytokine production data documented in IEDB shows that JanusMatrix analysis of epitope humanness differentiates between cytokine-positive and –negative data of regulatory and effector T cell cytokines. Peptides documented as IL-10-positive have significantly higher potential for human T cell cross-reactivity. Peptides documented as IL-4-positive have significantly lower potential for human T cell cross-reactivity.
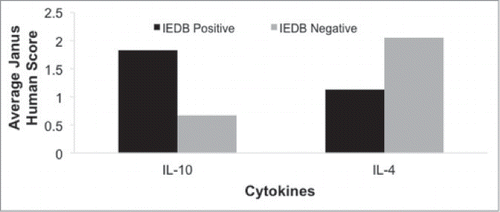
Figure 6. EpiMatrix protein immunogenicity scale. EpiMatrix protein immunogenicity scores higher than +20 are considered to be potentially immunogenic. On the left of the scale are well-known proteins for comparison. Low-scoring proteins near the bottom of the scale are known to engender little to no immunogenicity while higher scoring proteins near the top of the scale are known immunogens.
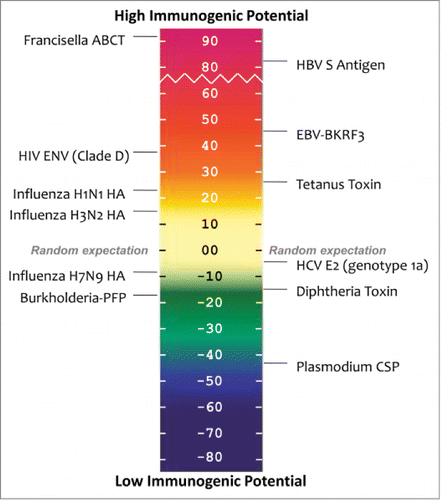
Figure 7. Potential immunogenicity of avian H7 and seasonal influenza HA. Avian H7 HA proteins possess low immunogenic potential based on EpiMatrix-predicted CD4+ T cell epitope content. In contrast recent circulating seasonal HAs bear higher potential and fall within the positive range of the scale. Lower epitope content in H7N9 HA may explain, in part, lower H7N9 immunogenicity observed in infection and vaccination. Re-published from Hum Vaccin Immunother. 2014;10(2),256-62 with permission.
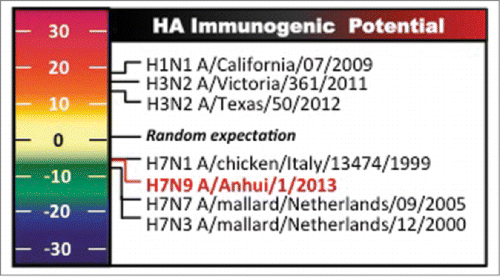