Figures & data
Figure 1. Fundamental components of materials science and engineering with (i) engineering focus and (ii) science focus [Citation3].
![Figure 1. Fundamental components of materials science and engineering with (i) engineering focus and (ii) science focus [Citation3].](/cms/asset/03e70d05-86cf-4205-84b6-18ee9540696b/tmrl_a_2054668_f0001_oc.jpg)
Figure 2. Schematic diagram of energy landscape as a function of one internal process [Citation1].
![Figure 2. Schematic diagram of energy landscape as a function of one internal process [Citation1].](/cms/asset/f98bfb59-324d-4e1a-b61c-3a18fd293af9/tmrl_a_2054668_f0002_oc.jpg)
Figure 3. Relationships among tracer diffusivity, atomic mobility, kinetic L parameters, and intrinsic and chemical diffusivities.
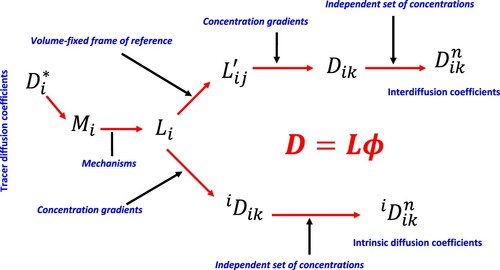
Figure 4. Calculated Seebeck coefficients for (i) PbTe for various p- and n-type doping levels [Citation68]; (ii) p-type SnSe [Citation68]; (iii) [Citation69].
![Figure 4. Calculated Seebeck coefficients for (i) PbTe for various p- and n-type doping levels [Citation68]; (ii) p-type SnSe [Citation68]; (iii) La2.75Te4 [Citation69].](/cms/asset/8e428f8f-e3bd-41d8-99f6-3b8c2fbd2355/tmrl_a_2054668_f0004_oc.jpg)
Figure 5. Signs of the Soret coefficients in the water (H2O), ethanol (ETH), and triethylene glycol (TEG) ternary system at 25 C [Citation96]. The colored regions denote regions of negative Soret coefficients of the respective components. Point Z marks the intersection of the boundaries of the three colored regions, where all three Soret coefficients vanish simultaneously. The steady state optical signal vanishes along the dashed line.
![Figure 5. Signs of the Soret coefficients in the water (H2O), ethanol (ETH), and triethylene glycol (TEG) ternary system at 25 C [Citation96]. The colored regions denote regions of negative Soret coefficients of the respective components. Point Z marks the intersection of the boundaries of the three colored regions, where all three Soret coefficients vanish simultaneously. The steady state optical signal vanishes along the dashed line.](/cms/asset/bf27062a-b37e-4405-8892-03a38890d492/tmrl_a_2054668_f0005_oc.jpg)
Figure 6. Simulated concentration profiles for 102 and 13,889 hours [Citation87] with experimental data from ref [Citation111] after 102 hours superimposed.
![Figure 6. Simulated C concentration profiles for 102 and 13,889 hours [Citation87] with experimental data from ref [Citation111] after 102 hours superimposed.](/cms/asset/8be6af76-9bb0-44f6-b679-08eec71f932b/tmrl_a_2054668_f0006_ob.jpg)
Figure 7. (i) Chemical potential of C in Fe-Si-0.45C alloys plotted with respect to the Si content at 1050°C with the TCFE9 thermodynamic database [Citation113]; (ii) C composition profile in diffusion couple I after 13 days; (iii) C and Si compositions in the diffusion couple I with the numbers used to calculate the distance from the high Si side with the formula shown in the diagram.
![Figure 7. (i) Chemical potential of C in Fe-Si-0.45C alloys plotted with respect to the Si content at 1050°C with the TCFE9 thermodynamic database [Citation113]; (ii) C composition profile in diffusion couple I after 13 days; (iii) C and Si compositions in the diffusion couple I with the numbers used to calculate the distance from the high Si side with the formula shown in the diagram.](/cms/asset/916dd2f3-0fc4-4530-ab23-4d38d18ca97c/tmrl_a_2054668_f0007_ob.jpg)
Figure 8. Chemical potential of C in the Fe-0.80C alloy is increased only slightly by the Co content at 1000°C, calculated using a CALPHAD thermodynamic database and Thermo-Calc software [Citation112,Citation113].
![Figure 8. Chemical potential of C in the Fe-0.80C alloy is increased only slightly by the Co content at 1000°C, calculated using a CALPHAD thermodynamic database and Thermo-Calc software [Citation112,Citation113].](/cms/asset/18ca0cd6-7db9-4ffd-bb76-eca4ea827ec8/tmrl_a_2054668_f0008_ob.jpg)
Table 1. Physical quantities related to the first directives of molar quantities (first column) to potentials (first row), symmetric due to the Maxwell relations [Citation1,Citation11,Citation345].
Table 2. Cross phenomenon coefficients represented by derivatives between potentials [Citation23].
Figure 9. Predicted phase diagrams of (i) temperature-pressure [Citation245] with symbols for experimental data and (ii) temperature-volume [Citation249] with purple diamond squares for thermal expansion anomaly and other symbols for experimental data.
![Figure 9. Predicted phase diagrams of Ce (i) temperature-pressure [Citation245] with symbols for experimental data and (ii) temperature-volume [Citation249] with purple diamond squares for thermal expansion anomaly and other symbols for experimental data.](/cms/asset/caa7a2d4-a3fe-4b7b-a288-1d916c1044ff/tmrl_a_2054668_f0009_oc.jpg)
Figure 10. Predicted phases diagrams of (i) temperature-pressure [Citation250] with experimental data superimposed, noting pressure decrease from left to right, and (ii) temperature-volume [Citation249] with experimental data (black circles) on the ambient pressure (0 GPa) volume curve and the purple diamonds for the anomalous regions of negative thermal expansions.
![Figure 10. Predicted phases diagrams of Fe3Pt (i) temperature-pressure [Citation250] with experimental data superimposed, noting pressure decrease from left to right, and (ii) temperature-volume [Citation249] with experimental data (black circles) on the ambient pressure (0 GPa) volume curve and the purple diamonds for the anomalous regions of negative thermal expansions.](/cms/asset/ca273370-28b4-4c74-8499-62991787cff8/tmrl_a_2054668_f0010_oc.jpg)
Figure 11. QCP phase diagrams: (i) temperature with respect to magnetic field of with antiferromagnetic (AF), non-Fermi liquid (NFL), and Landau Fermi liquid (LFL) phase regions [Citation213], (ii) temperature with respect to magnetic field of
and
with AF (blue left), NFL (yellow), and LFL (blue, right) phase regions [Citation214], (iii) temperature with respect to pressure with contour map of electrical resistivity of Al-doped CrAs and pure CrAs [Citation253], and (iv) temperature with respect to composition of
with contour maps for electrical resistivity (ρ) and a parameter (A*) related to quasiparticle effective mass [Citation255].
![Figure 11. QCP phase diagrams: (i) temperature with respect to magnetic field of YbRh2Si2 with antiferromagnetic (AF), non-Fermi liquid (NFL), and Landau Fermi liquid (LFL) phase regions [Citation213], (ii) temperature with respect to magnetic field of YbRh2Si2 and YbRh2(Si0.95Ge0.05)2 with AF (blue left), NFL (yellow), and LFL (blue, right) phase regions [Citation214], (iii) temperature with respect to pressure with contour map of electrical resistivity of Al-doped CrAs and pure CrAs [Citation253], and (iv) temperature with respect to composition of FeSe1−xSx with contour maps for electrical resistivity (ρ) and a parameter (A*) related to quasiparticle effective mass [Citation255].](/cms/asset/83f4fd12-13d9-4220-a4ed-320ac4e2baca/tmrl_a_2054668_f0011_oc.jpg)
Figure 12. Superconducting phase diagrams, (i) cuprates [Citation275], (ii) [Citation256], (iii)
[Citation276], and (iv) carbon- and hydrogen-doped
[Citation277].
![Figure 12. Superconducting phase diagrams, (i) cuprates [Citation275], (ii) CeM2X2 [Citation256], (iii) Ba(Fe1−xMx)2As2 [Citation276], and (iv) carbon- and hydrogen-doped H3S [Citation277].](/cms/asset/c187b525-afab-45bf-989f-d14dab28e8f4/tmrl_a_2054668_f0012_oc.jpg)
Figure 13. Orthorhombic- (i) SDW ordering temperature (
, red curve) and characteristic temperature (
, blue curve) plotted with respect to pressure with experimental data (symbols) [Citation282], and (ii) Fermi surface, Isosurfaces: (a) Stripe and (b) SDW; Cuts: (c) Stripe and (d) SDW; Top views of isosurfaces: (e) Stripe and (f) SDW [Citation281].
![Figure 13. Orthorhombic-BaFe2As2 (i) SDW ordering temperature (TSDW, red curve) and characteristic temperature (T∗, blue curve) plotted with respect to pressure with experimental data (symbols) [Citation282], and (ii) Fermi surface, Isosurfaces: (a) Stripe and (b) SDW; Cuts: (c) Stripe and (d) SDW; Top views of isosurfaces: (e) Stripe and (f) SDW [Citation281].](/cms/asset/71ffbe8f-f8a7-4b6a-beac-a458c8e1d518/tmrl_a_2054668_f0013_oc.jpg)
Figure 14. Phase diagrams of (i) and normalized intensity of the superstructure spots as a function of pressure in Hg-1201 [Citation303], (ii)
plotted against hole concentration in Hg-1201 with hole concentration for (i) marked by the vertical rectangle and various phase regions [Citation303], (iii)
plotted against pressure in
[Citation304] with various phase regions: SDW (see Figure (i)), Tetra (tetragonal), Ortho (orthorhombic), M (magnetic), Nematic (no magnetic long-range), and SC (superconducting).
![Figure 14. Phase diagrams of (i) TC and normalized intensity of the superstructure spots as a function of pressure in Hg-1201 [Citation303], (ii) T plotted against hole concentration in Hg-1201 with hole concentration for (i) marked by the vertical rectangle and various phase regions [Citation303], (iii) T plotted against pressure in FeSe1−xSx [Citation304] with various phase regions: SDW (see Figure 13(i)), Tetra (tetragonal), Ortho (orthorhombic), M (magnetic), Nematic (no magnetic long-range), and SC (superconducting).](/cms/asset/6e0521d5-69cf-4158-8a9b-7f889da2a3f0/tmrl_a_2054668_f0014_oc.jpg)
Figure 15. Gibbs energy diagram of an alloy system A-B with the effect of a driving force for diffusion acting over an interface between α and β, and the tangents describing the chemical potentials on both sides of the interface rotated relative to each other [Citation326].
![Figure 15. Gibbs energy diagram of an alloy system A-B with the effect of a driving force for diffusion acting over an interface between α and β, and the tangents describing the chemical potentials on both sides of the interface rotated relative to each other [Citation326].](/cms/asset/51c540b4-c1d9-4050-987b-450c4ab52fcc/tmrl_a_2054668_f0015_ob.jpg)
Figure 16. Dissolution of cementite at 910°C in an Fe-2.06Cr-3.91C (at. pct) alloy, (i) Isothermal section [Citation120], (ii) Cr concentration profile after 1000 s [Citation120], (iii) isothermal section with C activity plotted on the x-axis [Citation334], (iv) transmission electron microscope (TEM) bright field image of cementite exhibiting Widmanstatten plates of α-bcc phase (γ-fcc at dissolution temperature) [Citation334], and (v) TEM bright field image of extraction replica showing lamellar structure (
at point A) and untransformed
(darker area) [Citation334].
![Figure 16. Dissolution of cementite at 910°C in an Fe-2.06Cr-3.91C (at. pct) alloy, (i) Isothermal section [Citation120], (ii) Cr concentration profile after 1000 s [Citation120], (iii) isothermal section with C activity plotted on the x-axis [Citation334], (iv) transmission electron microscope (TEM) bright field image of cementite exhibiting Widmanstatten plates of α-bcc phase (γ-fcc at dissolution temperature) [Citation334], and (v) TEM bright field image of extraction replica showing lamellar M7C3 structure (uCr=0.54 at point A) and untransformed MC3 (darker area) [Citation334].](/cms/asset/e380dc03-bda1-427b-b1c3-d39c29a384e8/tmrl_a_2054668_f0016_ob.jpg)
Figure 17. Classification schemes of kinetic processes in relation to fundamental components of materials science and engineering.
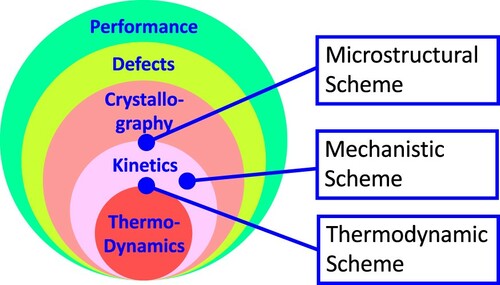