Figures & data
Table 1. Major eukaryotic translation initiation factors and their functions
Figure 1. Schematic depiction of translation initiation processes (key steps) in prokaryotes and eukaryotes (for eukaryotes, the canonical/scanning pathway is shown). See text for details.
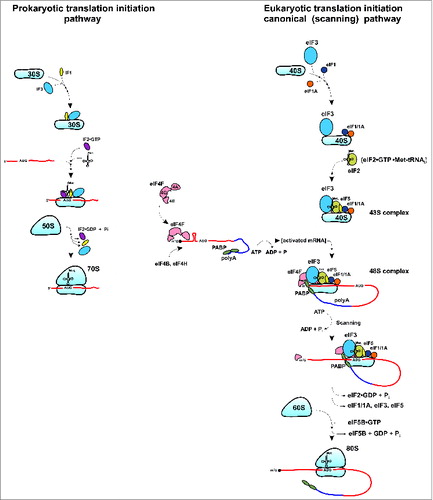
Figure 2. Graphical representation of the conservation of small ribosomal subunit protein families between different domains of life (E: Eukarya, A: Archaea, and B: Bacteria; left pie chart). Fifteen protein families are conserved across all domains (EAB) and 13 of these 15 conserved families have evolved eukaryote-specific extensions (right pie chart).
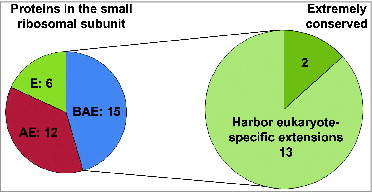
Figure 3. Ribosomal small subunit protein families with eukaryote-specific extensions. (A) Pie chart depicting the distribution of eukaryote-specific extensions (N-terminal, C-terminal, etc.) relative to the conserved part of the protein for the 13 ribosomal small subunit proteins with known extensions (based on the structural information available for the yeast and bacterial ribosomesCitation5,6,12,76,77). (B) Relative lengths of eukaryote-specific extensions in different conserved ribosomal small subunit proteins based on structural alignment of yeast (S. cerevisiae) and bacterial proteins. Blue bars depict N-terminal extensions, red bars depict C-terminal extensions and yellow bars show the length of the entire protein (in the yeast S. cerevisiae for all). (C) Variability in the relative lengths of eukaryote-specific extensions in different conserved ribosomal small subunit proteins based on alignment of S. cerevisiae, Schizosaccharomyces pombe, Mus Musculus, Homo sapiens and Xenopus laevis sequences (relative to corresponding E. coli sequences). Average lengths for the listed eukaryotic species are shown, with standard deviations indicated by error bars. Blue bars depict N-terminal extensions, red bars depict C-terminal extensions and yellow bars show the length of the entire protein. New (universal) nomenclature of ribosomal proteins is used.Citation49
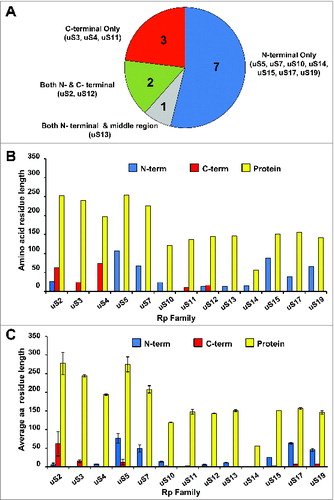
Figure 4. Ribosomal location of eukaryote-specific extension segments of conserved proteins from the small subunit and their known, or potential, functional significance. Only eukaryote-specific segments of conserved protein families are shown. New (universal) nomenclature of ribosomal proteins is used.Citation49 Yeast ribosomal protein names are provided in parentheses. Top – solvent side view. Bottom – interface side view. Extensions are color coded, with the rest of the protein content shown in cyan and rRNA content shown in gray. PDB entries 3U5GCitation6 and 3U5FCitation6 were used to build the models.
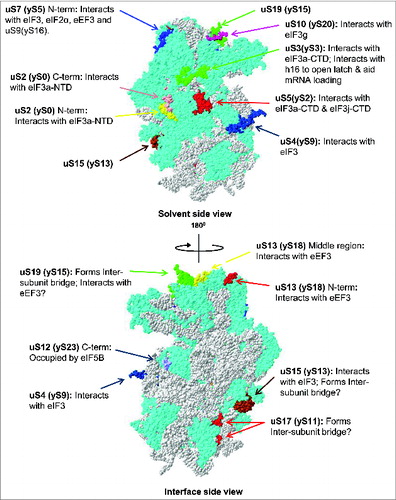
Figure 5. Sequence alignment of uS7 proteins from different organisms showing length variability of eukaryote-specific N-terminal extensions. uS7 protein sequences from fungi (S. cerevisiae, Candida glabrata, Ashbya gossypii, Neurospora crassa), fruit fly (D. melanogaster), worm (Caenorhabditis elegans), plant (Arabidopsis thaliana), vertebrates (Xenopus laevis, Mus musculus, Homo sapiens) and bacteria (E. coli) were aligned using the Clustal W algorithm. S. cerevisiae rpS5 (UniProtKB accession no.: P26783); C. glabrata rpS5 (UniProtKB accession number: Q6FSH6); A. gossypii rpS5 (UniProtKB accession no.: Q75D52); D. melanogaster rpS5 (UniProtKB accession number: Q24186); N. crassa rpS5 (UniProtKB accession no.: Q7RVI1); C. elegans rpS5 (UniProtKB accession number: P49041); A. thaliana rpS5 (UniProtKB accession no.: Q9ZUT9); H. sapiens rpS5 (UniProtKB accession number: P46782); M. musculus rpS5 (UniProtKB accession no.: P97461); X. laevis rpS5 (UniProtKB accession number: Q7SYU3); E. coli rpS7 (UniProtKB accession no.: Q0TCB8) sequences were used.
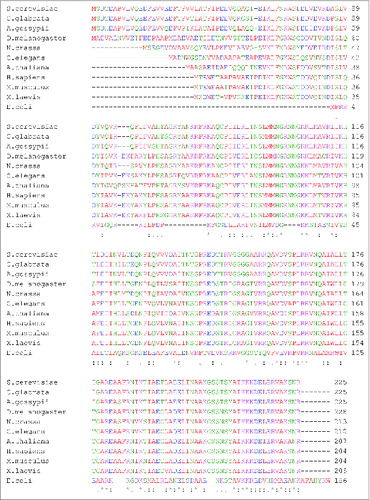
Table 2. Conserved proteins from the 40S small ribosomal subunit with eukaryote-specific extensions: interacting partners
Figure 6. Ribosomal location of eukaryote-specific segments of conserved proteins from the small subunit relative to bound eIF3, ternary complex (TC) and eIF5B. Eukaryote-specific extensions are shown in red, all other protein moieties are in cyan and rRNA is in gray. The location of mammalian eIF3 densitiesCitation30 (shown in orange) and archaeal TCCitation30 (shown in light blue) are traced onto the 40S surface. The putative location of eIF5BCitation15 is shown in pink.
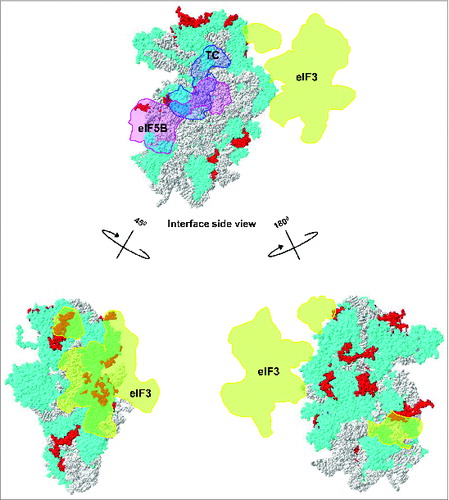
Figure 7. Ribosomal location of conserved proteins uS3, uS7, uS9. (A) The eukaryote-specific C-terminal extension of uS3 interacts with Asc1/RACK1. The eukaryote specific region of uS3 is shown in red with the rest of the protein shown in blue. Regions of Asc1/RACK1 interacting with the uS3 C-terminus are shown in pink with rest of RACK1 in yellow. (B) Ribosomal locations of uS7 and uS9. The eukaryote-specific N-terminal extension of uS7 is shown in red with rest of the protein in blue. uS9 is shown in yellow, with the region interacting with uS7 N-terminus in pink. PDB entry 3U5G was used.
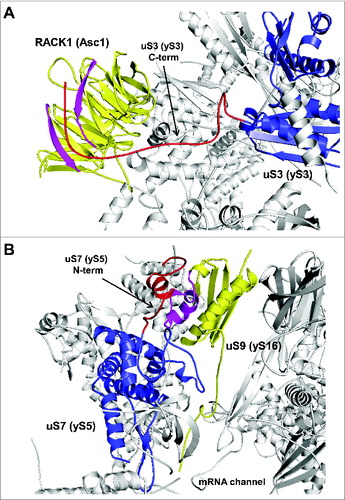
Figure 8. Ribosomal location of conserved proteins uS5 and uS11. (A) uS5 is located in the vicinity of the mRNA entry channel. The eukaryote-specific N-terminal extension forming a β-hairpin loop protruding into the mRNA entry channel is in red and the conserved protein moiety is shown in blue. (B) Ribosomal location of uS11. The eukaryote-specific C-terminal extension of uS11 protruding toward the mRNA exit channel is shown in red and the conserved protein moiety is shown in blue.
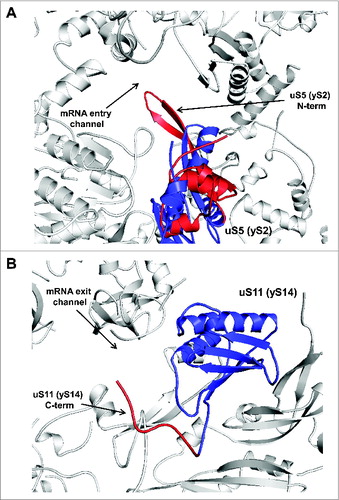