Figures & data
Table 1. Comparisons of CRISPR/Cas-based detection with current pathogen nucleic acid diagnostic methods.
Figure 1. Detection of pathogen nucleic acids with CRISPR-Cas9 based assay. (A) Schematic of NASBACC detection. A synthetic trigger sequence and T7 primer are appended to a NASBA-amplified RNA fragment through reverse transcription. The Cas9 specific cleavage of the sequence with PAM, leading to the production of either truncated or full-length trigger RNA, which differentially activates a toehold switch sensor. (B) Schematic of CAS-EXPAR detection. The Cas9/sgRNA complexed with designed PAMer induces site-specific cleavage of ssDNA substrates producing cleaved fragments. The fragment will be hybridized with the EXPAR template and amplified by DNA polymerase, which can be monitored with a real-time fluoresces. (C) Schematic of ctPCR detection. The CRISPR-typing PCR (ctPCR) integrates Cas9 cutting with PCR assay. By using Cas9 specific cleavage to get a second-round primer then start the amplification with PCR or qPCR, and visualized with gel or fluorescence readout. (D) Schematic of CASLFA detection. The CASLFA system means CRISPR/Cas9-mediated lateral flow nucleic acid assay. The CRISPR/Cas9 is integrated with a lateral flow detection platform, which will show a band on the test line of the strip when there appears the target sequence. (E) Schematic of Paired dCas9 (PC) reporter system detection. The firefly luciferase (NFluc or CFluc) is split and fused to two dCas9s. When the two dCas9s closely binding to the target sequence, will bring the half luciferase into proximity to form integral enzyme which catalyzes the bioluminescent reaction. (F) Schematic of CRISPR-mediated DNA-FISH detection. In the system, a magnetic nano-bead is fused with dCas9, and the target nucleic acid once bind by the dCas9-sgRNA complex will be isolated by magnetic and give the fluorescence signal by SYBR Green staining. (G) Schematic of CRISPR-Chip detection. CRISPR–Chip is composed of a gFET construct, on which the dCas9 complexed with a target-specific sgRNA immobilized on the surface of the graphene. When there is target DNA on the chip, the dCas9 kinetically binds to the target DNA, which will modulate the electrical characteristics of the gFET and result in electrical signal output. ssRNA, single-strand RNA; NASBA, nucleic acid sequence-based amplification; dsDNA, double-stranded DNA; PAM, protospacer-adjacent motif; ssDNA, single-stranded DNA; dCas9, Nuclease-deactivated Cas9; PC, paired dCas9; RCA, rolling circle amplification.
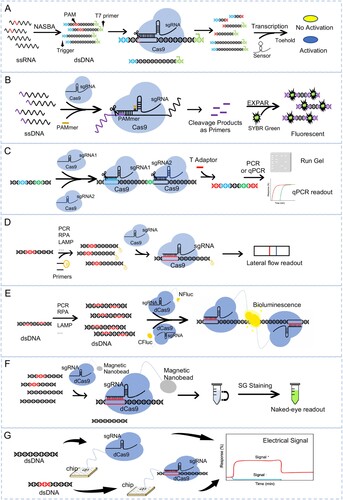
Figure 2. Diagnostic of pathogen nucleic acids with trans-cleavage of active CRISPR-Cas. Schematic of a CRISPR effector nuclease exploited for nucleic acid detection in SHERLOCK (Cas13a), DETECTR (Cas12a) or Cas4-DETECTR (Cas14a). In the absence of its nucleic acid target, the Cas nuclease is inactive. Upon binding of its guide crRNA to a cognate target (RNA for Cas13a, dsDNA for Cas12a, ssDNA for Cas14a), the nuclease is activated, leading to target cleavage and catalytic cleavage of nearby nucleic acids (ssRNA for Cas13a, ssDNA for Cas12a and Cas14a). This “collateral” nuclease activity is turned into an amplified signal by providing reporter probes with a fluorophore (usually FAM) linked to a quencher (or Biotin for later flow assay) by a short oligonucleotide. Upon cleavage of the reporter by the activated nuclease, the reporter nucleotide will break and thus fluoresces bright or band appearing in a strip. In both SHERLOCK and DETECTR, target abundance is enhanced by isothermal pre-amplification using RPA with or without in vitro transcription or reverse transcription.
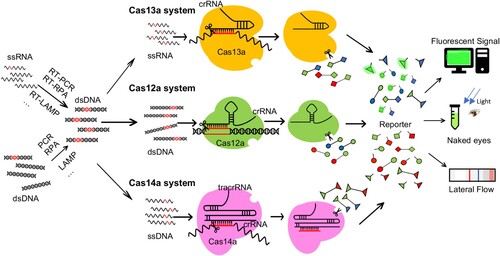
Table 2. Diagnostic of infection pathogen with CRISPR/Cas-based detection.
Figure 3. Pathogen diagnostic and alarm with CRISPR/Cas-based cloud detection. Schematic diagram of an early alarm of infection risk system based on CRISPR-Cas rapid diagnostic and artificial intelligence (AI) powered predication. Individual patient from the community, hospital or health centre, can be rapidly and accurately detected with the specific infectious pathogen. The readout data will be transformed into the cloud for store and process through the 5G service of a mobile phone app. The cloud computing will calculate the infectious risk to generate an AI-powered model on update diagnostic cases, then warn the administrators or individuals.
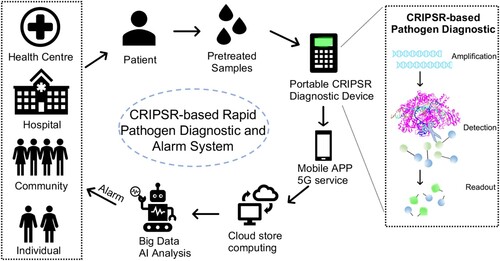