Figures & data
Figure 1. General 1:500 000 geological map across the southern Nagssugtoqidian Orogen simplified from Garde and Marker (Citation2010). The location of number of mafic and ultramafic complexes mapped by processing of regional HyMAP data are shown in pink ovals. The Niaqornarssuit complex is highlighted by a black oval
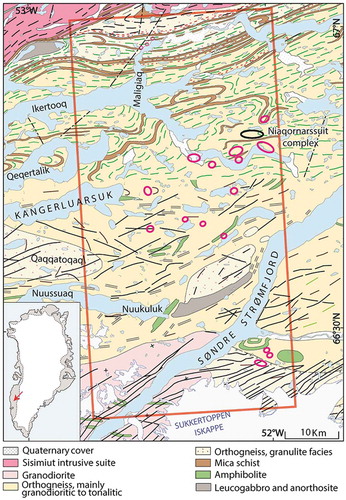
Figure 2. View of the Niaqornarssuit complex, highlighting characteristic features of the study area terrane (e.g. variability in spatial continuity of exposed outcrop, lichen coatings, and low-lying vegetation cover)
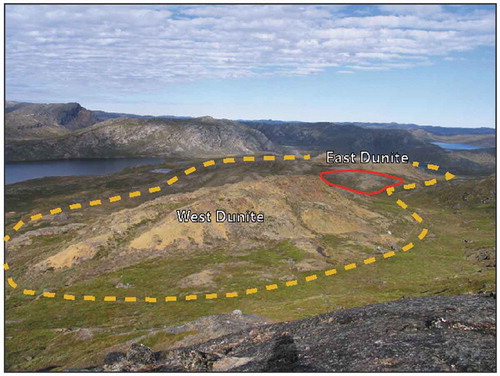
Figure 3. True color HyMAP image and detailed geological map of the Niaqornarssuit complex produced by 21st North Exploration Company. Yellow dots show the locations of surface samples that have been collected for reconnaissance work and mapping over the area. Samples that are selected for XRF and ASD analysis are indicated by red color
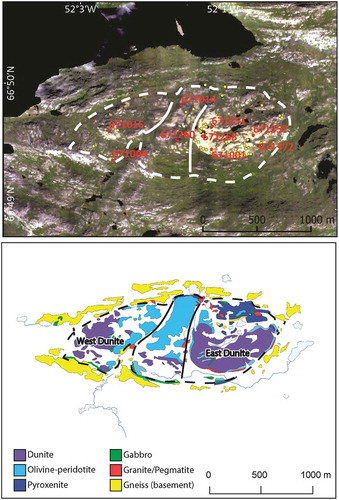
Figure 4. (a) Field localities where spectroradiometric measurements were carried out. The area covered by the airborne hyperspectral survey is indicated by the red line. K = kimberlitic rocks, L = lamproitic rocks, LG = local geology, S = rocks of the Sarfartoq carbonatite complex, P = pseudo-invariant fields, Plant = vegetation; (b) locality P3 on the silt formation in the vicinity of Kangerlussuaq airport; (c) individual measurements from the locality P3
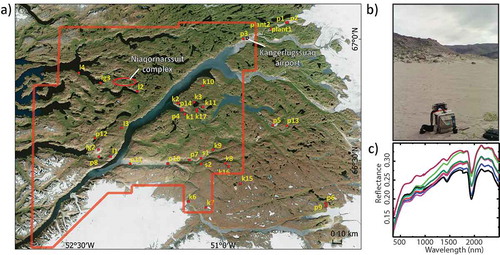
Figure 5. Airborne HyMAP data before and after removal of remaining albedo differences using detrending approach
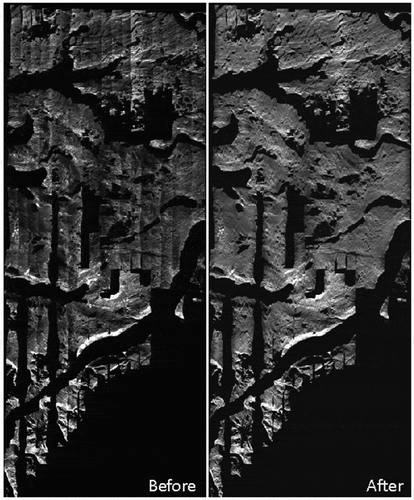
Table 1. Mafic and ultramafic samples and mineralogical composition determined from X-ray fluorescence analysis (hornblende: hnb, pyroxene: px, olivine: ol, serpentine: srp)
Figure 7. Spectral end-members used for ISMA and EnGeoMAP mapping methods. Outcrop related endmembers using (a) full range of spectral bands and (b) knowledge-based band selection. Vegetation and lichen related endmembers using (c) full range of spectral bands and (d) knowledge-based band selection. See for detailed description of the selected spectral bands
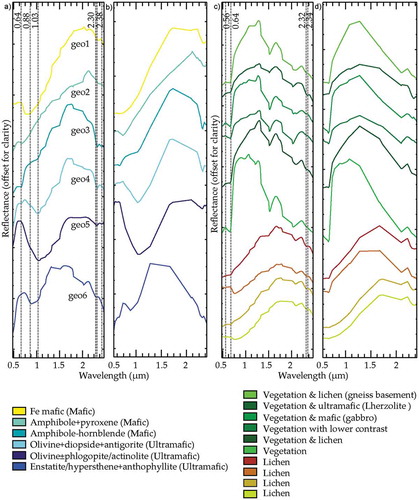
Figure 8. Comparison of HyMAP endmembers (solid lines) derived from SSEE with best matches from laboratory sample average spectra and mineral spectra taken from the USGS spectral library for (a,b) gabbro, (c,d) pyroxenite, (e,f) peridotite and (g,h) dunite samples. See for detailed description of mineralogical composition of each sample determined from X-ray fluorescence analysis
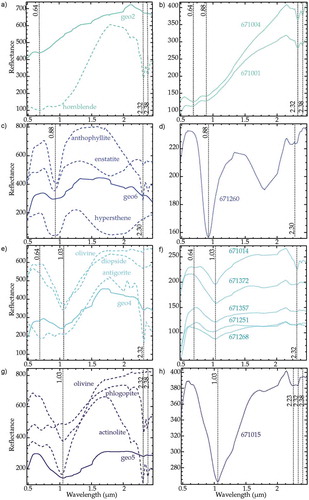
Table 2. Spectral bands used for SSEE and ISMA, wavelength in µm
Figure 9. (a) HyMAP continuum removed RGB color composite highlighting the main mafic-ultramafic units. Red – ferric iron (0.64 μm); Green – Mg-OH (2.32 μm); and Blue – ferrous iron (1.03 μm). (b) False-colour composite image highlighting the abundance of vegetation in the area. Classification results for HyMAP data generated from (c) ISMA, (d) best fit and (e) BVLS methods
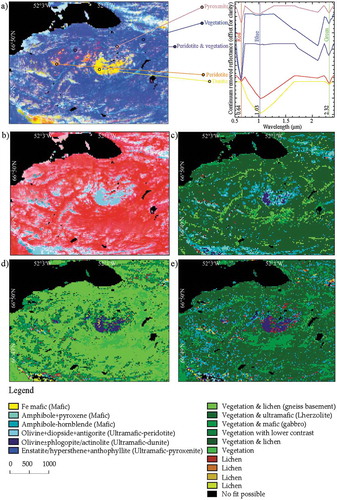
Figure 10. Spectral unmixing results from ISMA and EnGeoMAP showing abundances for (a,g) geo2 – mafic (amphibole + pyroxene); (b,h) geo3 – mafic (amphibole – hornblende); (c,i) geo4 – peridotite (olivine + diopside + antigorite); (d,j) geo5 – dunite (olivine ± phlogopite/actinolite); and (e,k) geo6 – pyroxenite (enstatite/hypersthene + anthophyllite) endmembers. RGB color composites of abundances for (f) ISMA and (l) EnGeoMAP highlighting the main mafic-ultramafic units. Red – mafic (geo2); Green – peridotite; and Blue – dunite
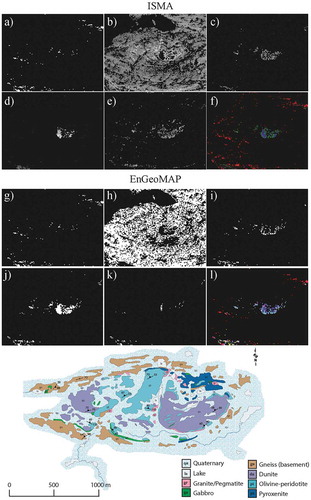
Figure 11. Classification results generated from BVLS for simulated ASTER, Landsat-8 OLI, and Sentinel-2. HyMAP BVLS and ISMA data are used as a reference base and similarity scores for each endmember and sensor to the HyMAP reference are indicated in the plot
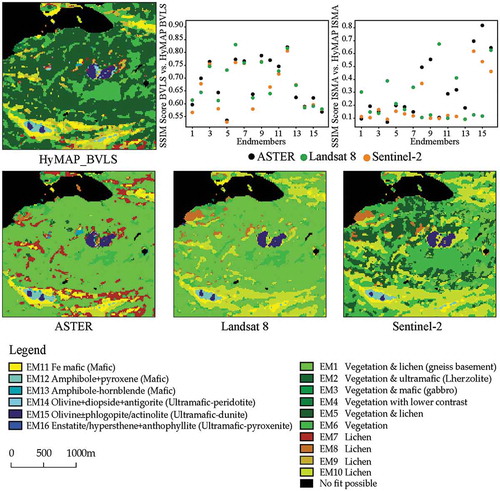
Table 3. Quantitative comparison between the results generated using different processing approaches with the reference image
Figure 12. a) The binarized dunite class from the geological map is used as ground truth. The binarized classification results for HyMAP data generated using b) ISMA, c) best fit, and d) BVLS methods for the dunite endmember
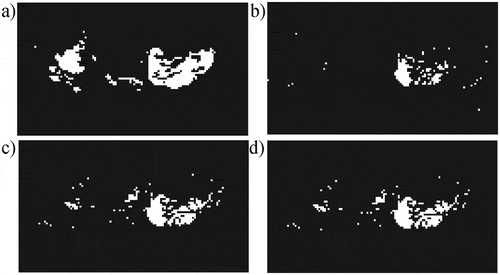