Figures & data
Table 1. Simulation parameters, see also for the definitions of lengths.
Figure 1. Rail deflection and bending moment due to a train passage. Camera A measures the undeformed rail surface while Camera B measures the deformed surface. The dimensions ,
, and
are described in , and
is the distance from the wheel to Camera B.
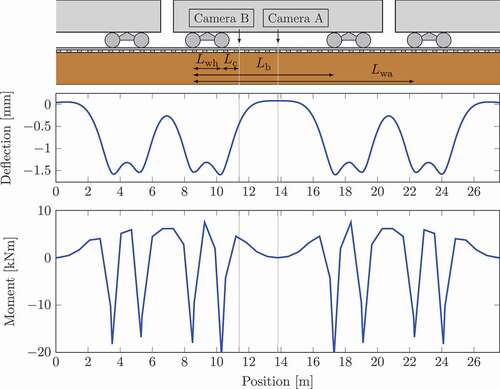
Figure 2. Illustration of the measured strain around a crack mouth in a rail subjected to a positive bending moment.
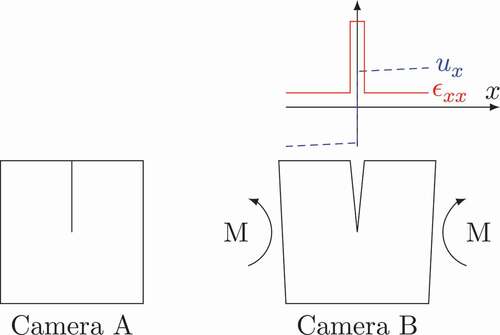
Figure 3. DIC experimental setup with the yellow hydraulic cylinders exerting a 4-point bending in the rail samples.
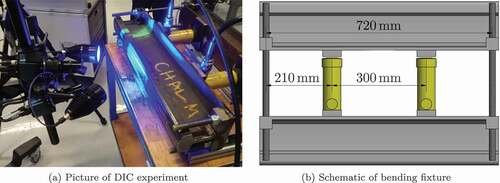
Figure 6. Example of conversion from optical microscopy images to binary images. The shown images are .
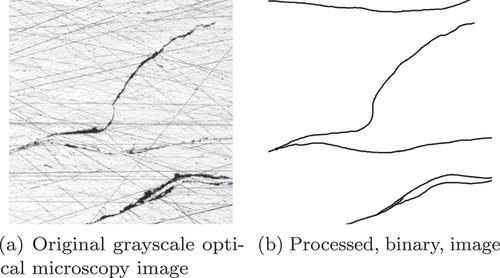
Figure 7. DIC results, viewed from above the microscopy sections. The black rectangle at shows the location of the reference mark, and axes correspond to the axes in . with dimensions in mm. The same strain scale as in ) is used. The coloured circles show the end-points of the cracks marked with the corresponding translucent colour in
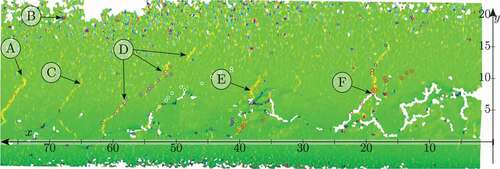
Figure 9. Sections showing the crack patterns with inverted colours compared to . All coordinates are in mm. The horizontal lines have 5 mm spacing, the numbers next to the positive and negative y-axes denote the respective axis’ length, and the -coordinate is defined in ). The translucent colours show how different cracks evolve between the sections and their top endpoints are marked by circles of the corresponding colour in
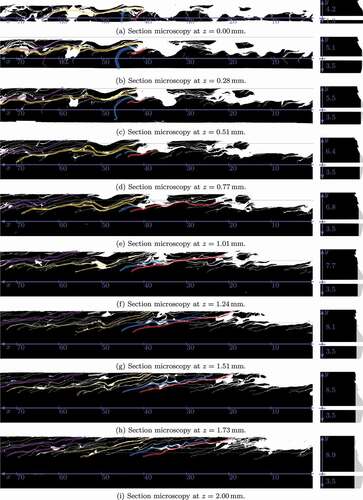
Figure 10. Correlation between cracks and DIC surface strains at . More sections are available in the supplementary material ‘CracksToDIC_Correlation.pdf’.
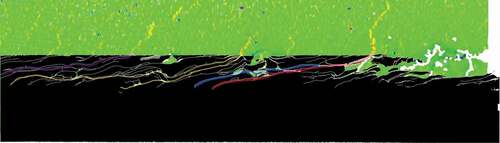