Figures & data
Figure 3. Predictions of (a) axial velocity, (b) radial velocity, (c) velocity at pipe exit and (d) axial temperature, for 50, 100, and 180 K mesh sizes showing meshing independence.
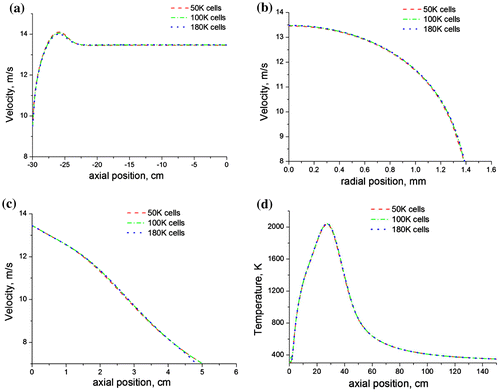
Figure 4. Axial predictions of (a) turbulent kinetic energy, (b) turbulent dissipation rate and (c) turbulent intensity for a methane–air jet diffusion flame at Re = 4,221 using the three turbulence models.
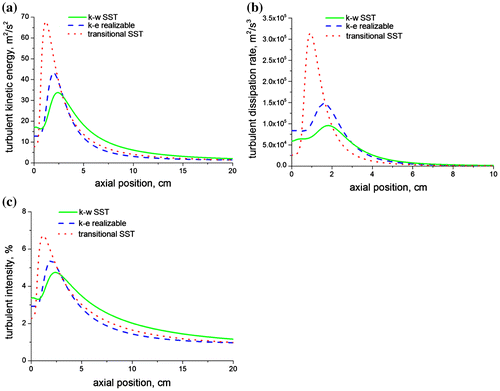
Figure 5. Radial predictions of the mean mixture fraction at (a) x/di = 9, (b) x/di = 60, and (c) x/di = 170 for a methane–air jet diffusion flame at Re = 4,221 using the three turbulence models.
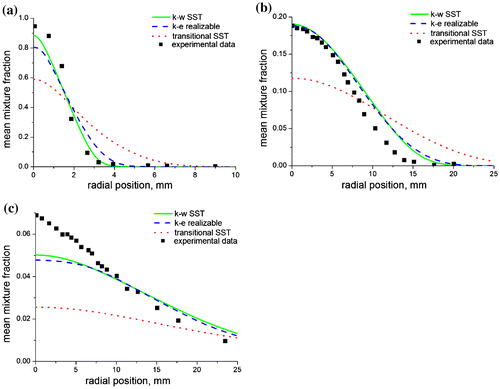
Figure 6. Radial predictions of temperature at (a) x/di = 9, (b) x/di = 60, and (c) x/di = 170 for a methane–air jet diffusion flame at Re = 4,221 using the three turbulence models.
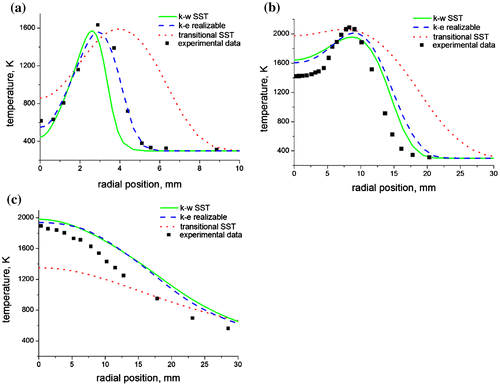
Figure 7. Radial predictions of the nitrogen concentrations at (a) x/di = 9, (b) x/di = 60, and (c) x/di = 170 for a methane–air jet diffusion flame at Re = 4,221 using the three turbulence models.
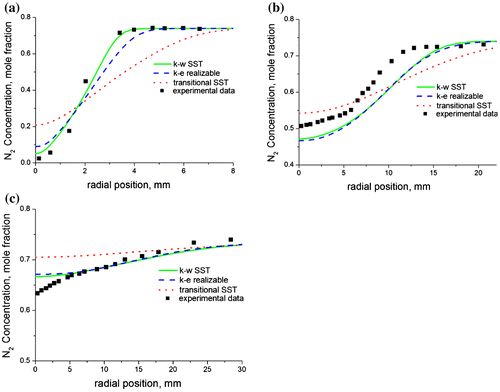
Figure 8. Radial predictions of the carbon dioxide concentrations at (a) x/di = 9, (b) x/di = 60, and (c) x/di = 170 for a methane–air jet diffusion flame at Re = 4,221 using the three turbulence models.
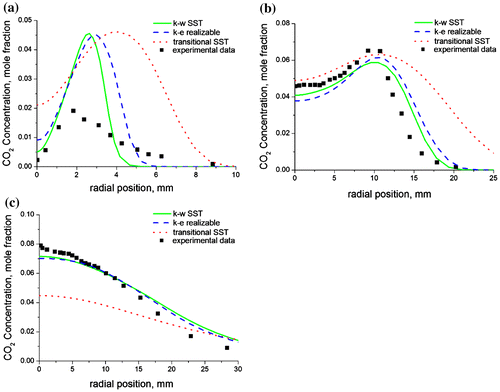