Figures & data
Figure 1. Global failure mechanisms of masonry structures.
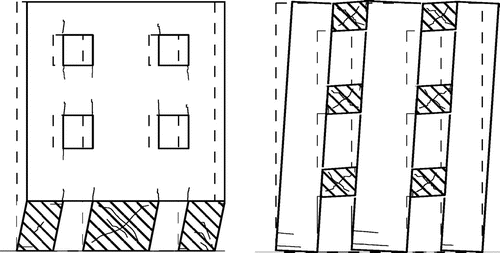
Figure 2. Observed out-of-plane failure modes for masonry structures (D’Ayala & Speranza, Citation2003).
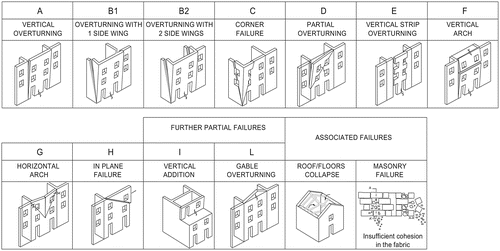
Figure 3. Damage mechanisms of rubble stone masonry.
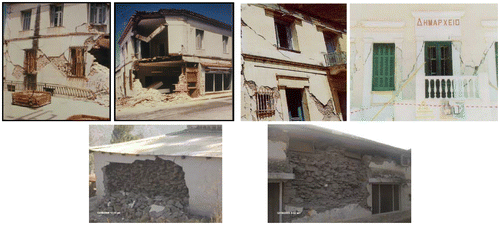
Figure 4. Graphical representation of DBELA.
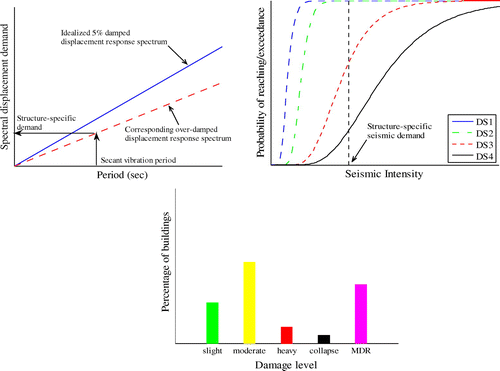
Figure 5. Nonlinear static SDOF idealization and capacity curve, mechanical model, for global mechanism.
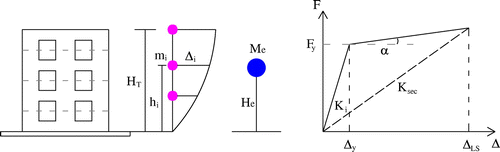
Figure 6. Nonlinear static SDOF idealization, mechanical model, of masonry wall for out-of-plane failure modes, after Doherty et al. (Citation2002).
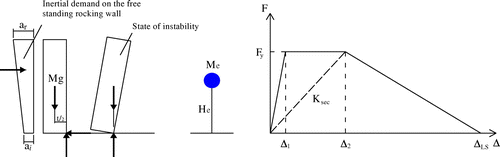
Figure 7. Flow chart for the derivation of vector-based displacement-based fragility functions, global mechanism.
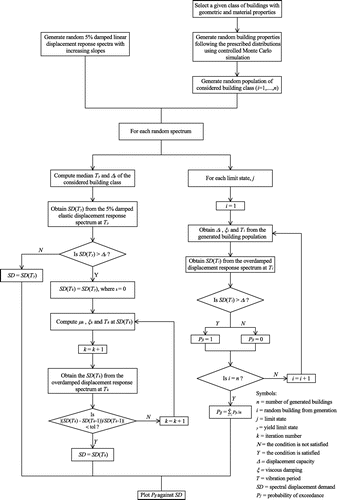
Figure 8. Flow chart for the derivation of vector-based displacement-based fragility functions, local mechanism.
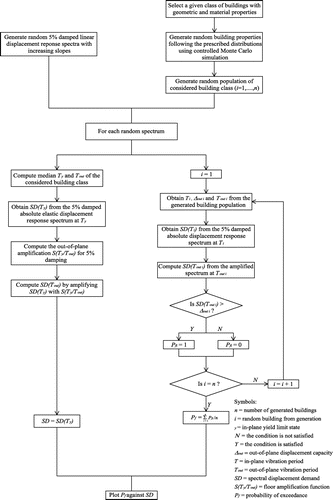
Figure 9. Definition of seismic demand on the out-of-plane walls.
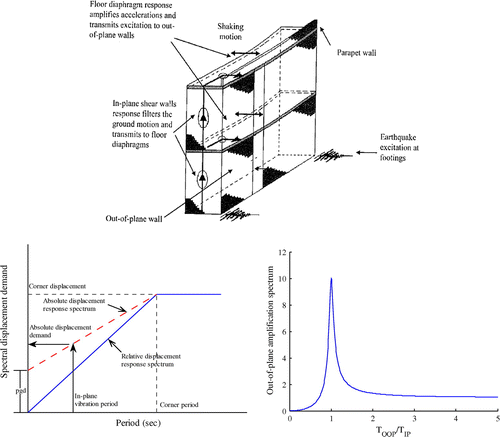
Figure 10. Equivalent frame method.
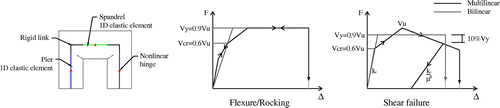
Figure 11. Lateral force-displacement response of case study masonry walls.
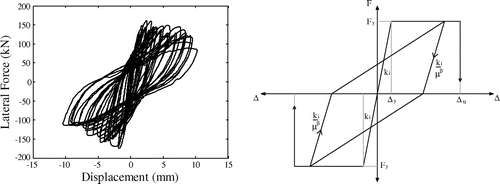
Figure 13. Validation of the proposed modelling hypothesis for masonry structure, tested at the University of Pavia (Magenes et al., Citation1995).
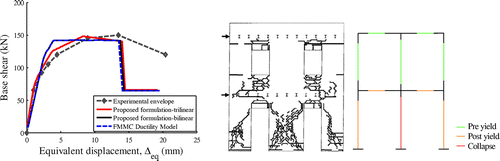
Figure 14. The effect of different wall density and floor area on the yield strength, exemplificative chart.
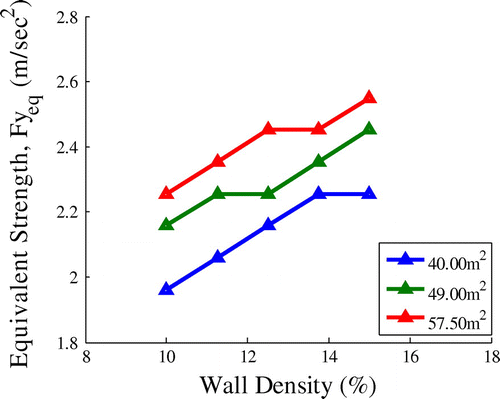
Table 1. Structural properties considered to generate proto type structural models for masonry structures
Figure 15. Pushover curves for case study two-storey low-rise structures and the derivation of capacity curves parameters.
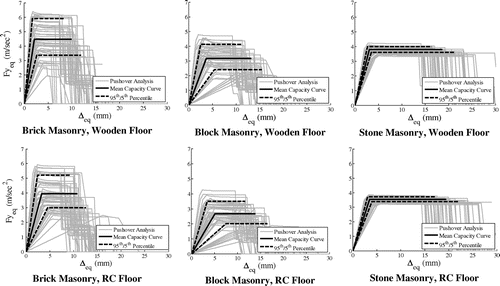
Table 2. Capacity curve parameters (median, lower and upper bound) for case study masonry structures
Figure 16. Mean spectrum of the selected accelerograms and comparison with the EC8 Type I-C soil spectrum.
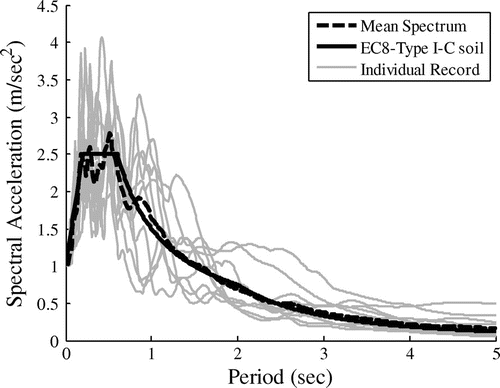
Table 3. Drift limits computed for case study masonry structures, in percent
Figure 17. Collapse multiplier for typical out-of-plane failure of façade walls obtained experimentally (Restrepo-Velez & Magenes, Citation2009).
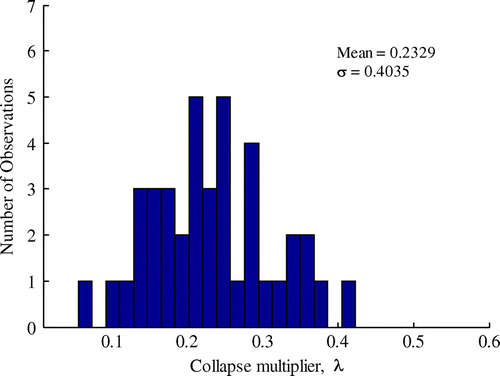
Table 4. Parameters used in the random generation of structures for fragility functions derivation for South-Asia countries
Figure 18. Vector-based fragility functions for case study masonry structures.
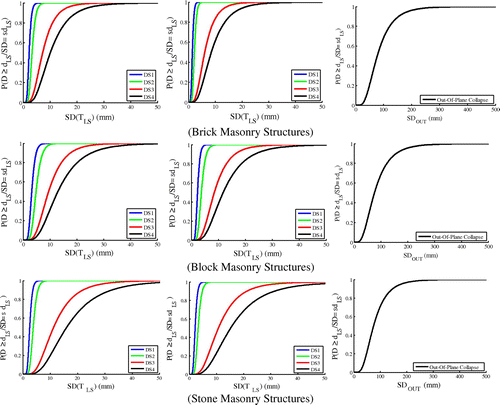
Figure 19. Scalar-based, SA (0.30 s), fragility functions for case study masonry structures.
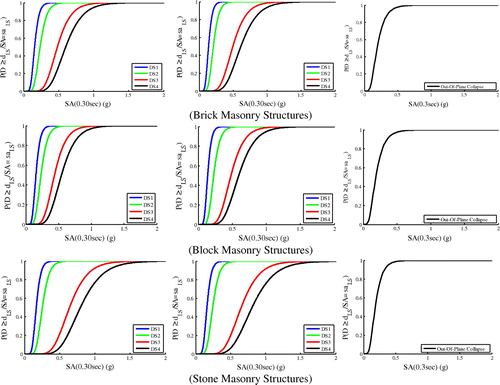
Figure 20. Scalar-based, PGA, fragility functions for case study masonry structures.
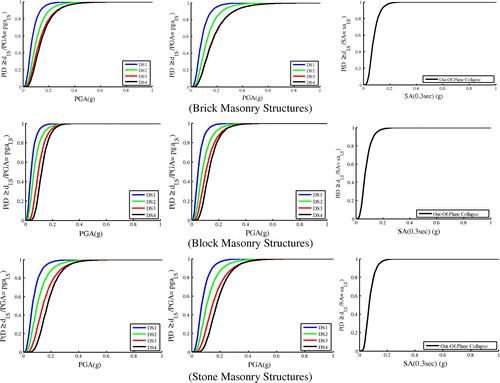
Figure 22. Percentage of structures damaged during 2005 Kashmir earthquake.
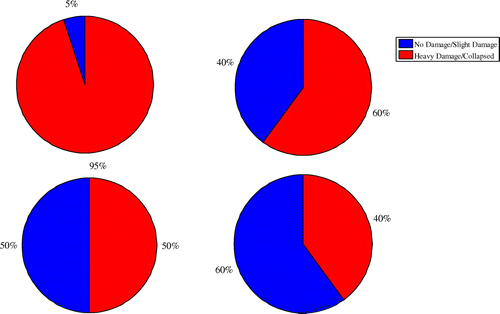
Table 5. Input parameters for vector-based (inelastic spectral displacement) fragility functions, lognormal distribution is conservatively considered
Table 6. Input parameters for scalar-based (elastic spectral acceleration), SA (0.30 s), fragility functions, lognormal distribution is conservatively considered
Table 7. Input parameters for scalar-based (peak ground acceleration) fragility functions, lognormal distribution is conservatively considered
Figure 23. Test and validation of the UPAV methodology against the 2005 Kashmir earthquake.
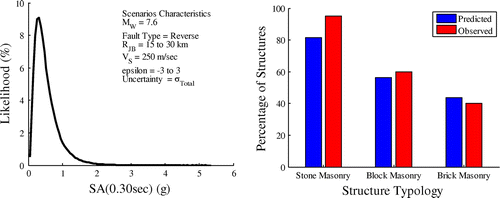