Figures & data
Figure 1. Channel geometry used for each simulation in this work. Boundary conditions: abcd: Cyclic; efgh: Cyclic; abfe: Cyclic; dcgh: Cyclic; bcgf: Wall; adhe: Wall
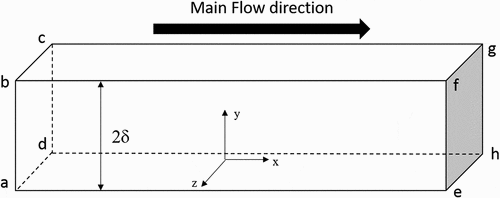
Table 1. Numerical schemes employed for all simulations performed in this work
Figure 2. Channel with Ribs-like protuberances. (a) Channel with ribs-like protuberances. Not all protuberances are shown. Dashed lines represent the protuberance for the SP position. (b) Details of the ribs-like protuberances. In total, five protuberances were set inside the computational box
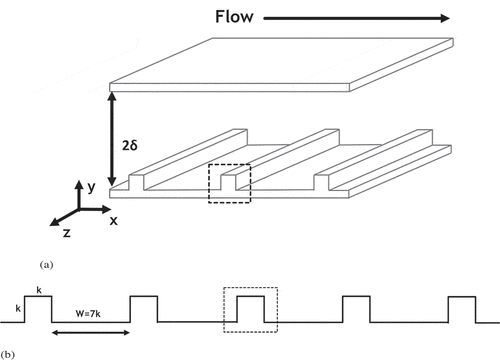
Figure 3. Channel with Cavity-like perturbations. (a) Channel with Cavity-like perturbations. Not all perturbations are shown. Dashed lines represent the perturbation where the SP position is located. (b) Details of the Cavity-like perturbations. In total, five perturbations were set inside the computational box
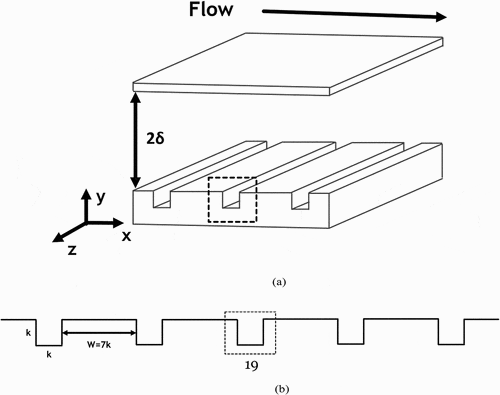
Table 2. Number of cells used for the smooth channel numerical experiments for each friction Reynolds number
Table 3. Grid resolution obtained for each simulation for the smooth channel cases
Table 4. Comparison of levels of grid resolution of simulations presented in this paper with similar works focused on turbulent channel flows at Reτ = 150. Grid resolution presented in inner wall units
Table 5. Number of cells used for the cases with Ribs-like protuberances and Cavity-like perturbations
Table 6. Nominal and actual Reτ values for each simulation
Table 7. k and C values for the logarithmic velocity profile approach for each simulation
Figure 4. Mean velocity profiles for each Reynolds number. Values of Mean velocity are normalised with uτ. DNS data were obtained from Lee & Moser (Citation2015) and Kim et al. (Citation1987)
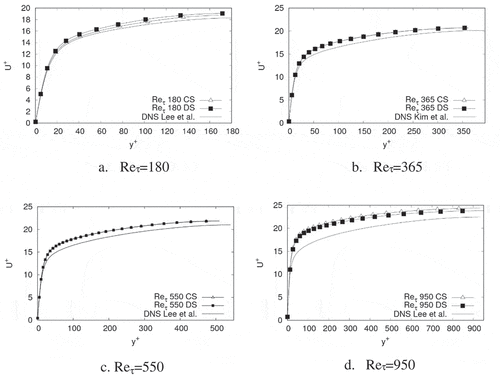
Figure 5. Mean velocity profiles for each Reynolds number. Mean velocity values are normalised with Ub. DNS data were obtained from Lee & Moser (Citation2015) and Kim et al. (Citation1987)
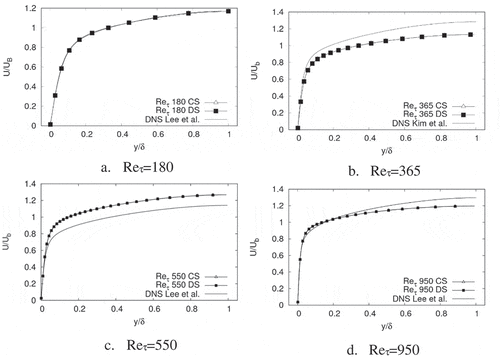
Figure 6. Mean velocity profiles for each Reynolds number. Mean velocity values are normalised with uτ. y+ coordinate is shown in log scale. DNS data were obtained from Lee & Moser (Citation2015) and Kim et al. (Citation1987)
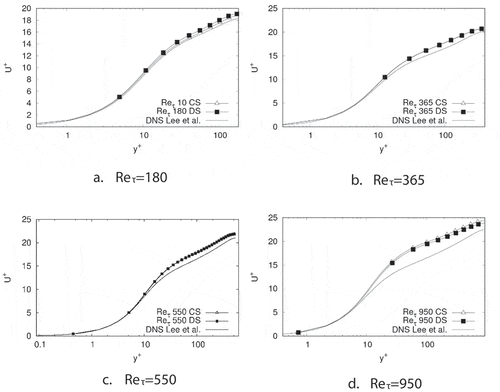
Figure 7. Mean velocity profiles for each Reynolds number. Mean velocity values are normalised with uτ. y+ coordinate is shown in log scale. Only sub-viscous layer is shown. DNS data were obtained from Lee & Moser (Citation2015) and Kim et al. (Citation1987)
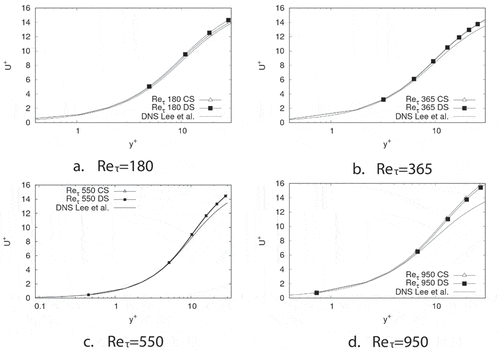
Table 8. Values of Cf obtained from each simulation and from Smooth-log profile and Dean’s Power-Law, respectively
Figure 9. Normalised turbulent kinetic energy for each Reynolds number and each SGS model. Turbulent kinetic energy is normalised with u2. DNS data were obtained from Lee & Moser (Citation2015) and Kim et al. (Citation1987)
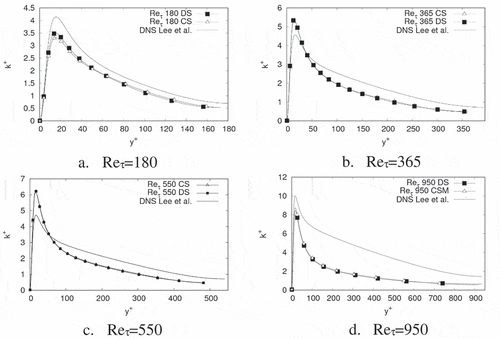
Figure 10. Root mean square (rms) values of velocity fluctuations in streamwise direction. Rms values are normalised with uτ. DNS values were taken from Lee & Moser (Citation2015) and Kim et al. (Citation1987). LES values were taken from Bose et al. (Citation2010) and Islam Mallik, Uddin, & Meah (Citation2014)
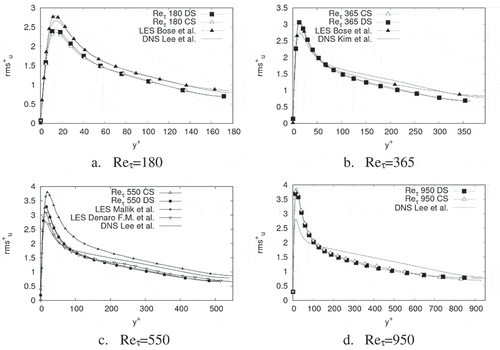
Figure 11. Root mean square (rms) values of velocity fluctuations in wall-normal direction. Rms values are normalised with uτ. Rms values are normalised with uτ. DNS values were taken from Lee & Moser (Citation2015) and Kim et al. (Citation1987). LES values were taken from Bose et al. (Citation2010) and Islam Mallik et al. (Citation2014)
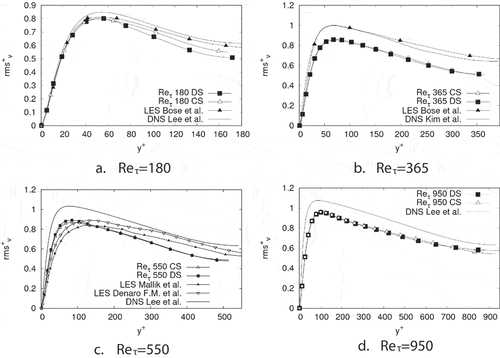
Figure 12. Root mean square (rms) values of velocity fluctuations in spanwise direction. Rms values are normalised with uτ. DNS values were taken from Lee & Moser (Citation2015) and Kim et al. (Citation1987). LES values were taken from Bose et al. (Citation2010) and Islam Mallik et al. (Citation2014)
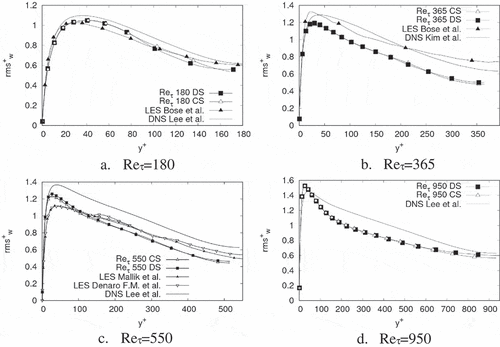
Figure 13. Relative error for rmsu. Relative errors are obtained by comparison between the simulations results for each Reynolds numbers and SGS models with available DNS data
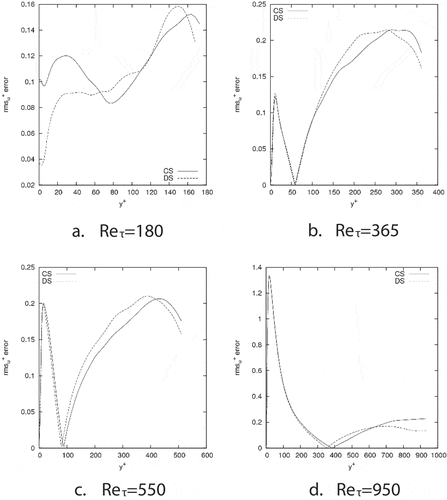
Figure 14. Relative error for rmsv. Relative errors are obtained by comparison between the simulations results for each Reynolds numbers and SGS models with available DNS data
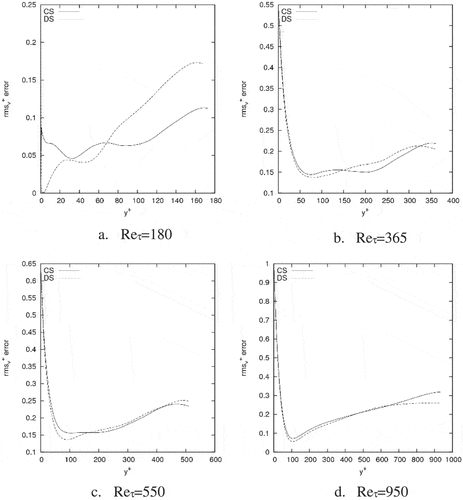
Figure 15. Relative error for rmsw. Relative errors are obtained by comparison between the simulations results for each Reynolds numbers and SGS models with available DNS data
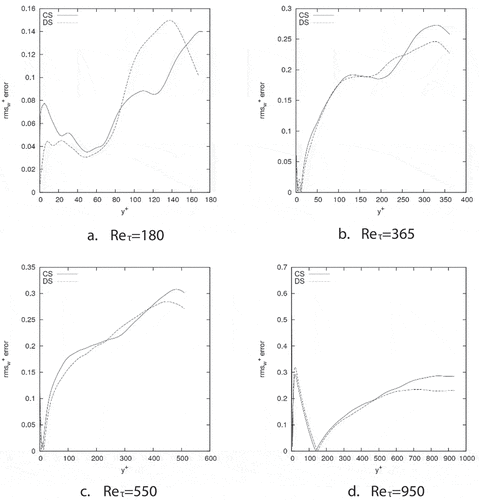
Figure 16. Maximun values of rms+ obtained for each Reynols number and SGS model. Comparison against DNS Data. DNS data were obtained from Lee & Moser (Citation2015) for Reτ = 180, 550, 950 and Kim et al. (Citation1987) for Reτ = 390
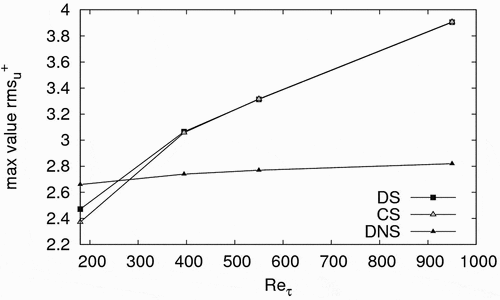
Figure 17. Relative error of the r.m.s values of the velocity fluctuations as a function of Reτ. Two positions over a vertical line normal to the walls are shown (y+ = 20 and y/δ = 1)
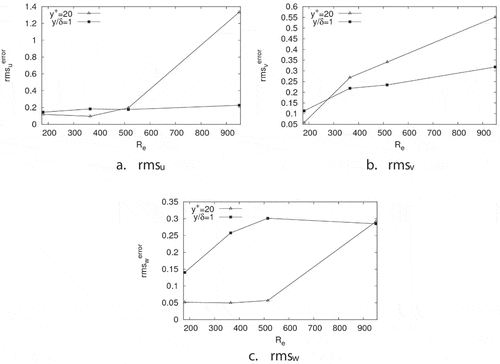
Figure 18. < u, v, >+ values. Data normalised with u2. DNS values were taken from Lee & Moser (Citation2015) and Kim et al. (Citation1987)
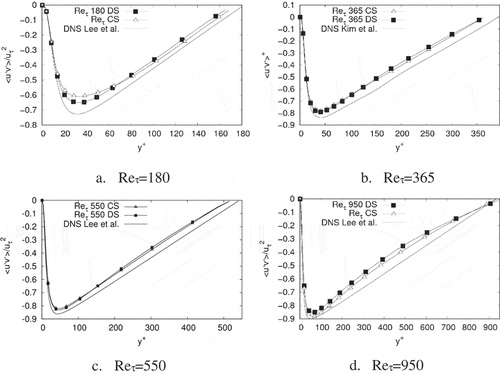
Figure 19. Coefficient of correlation between u, and v, for each Reynolds number and SGS model used in this work. DNS data were obtained from Lee & Moser (Citation2015) and Kim et al. (Citation1987)
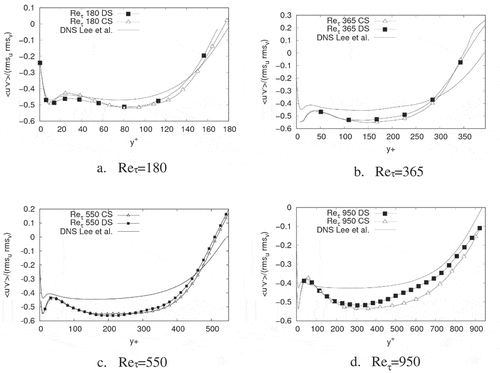
Figure 20. Turbulent production profiles for u, u, Reynolds stress tensor term for each Reynolds number and SGS model used in this work. DNS data were obtained from Lee & Moser (Citation2015) and Kim et al. (Citation1987)
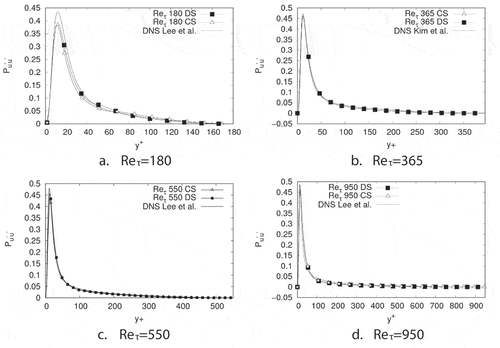
Figure 21. Turbulent production profiles for u, v, Reynolds stress tensor term for each Reynolds number and SGS model used in this work. DNS data were obtained from Lee & Moser (Citation2015) and Kim et al. (Citation1987)
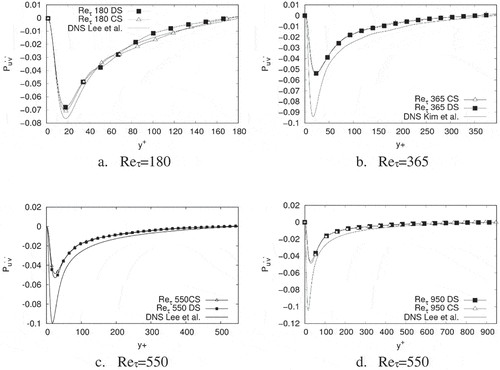
Figure 22. Viscous dissipation profiles for u, u, Reynolds stress tensor term for each Reynolds number and SGS model used in this work. DNS data were obtained from Lee & Moser (Citation2015) and Kim et al. (Citation1987)
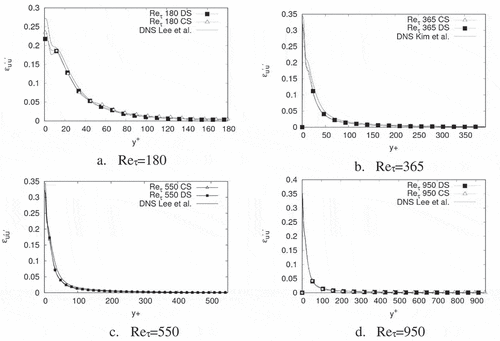
Figure 23. Viscous dissipation profiles for u, v, Reynolds stress tensor term for each Reynolds number and SGS model used in this work. DNS data were obtained from Lee & Moser (Citation2015) and Kim et al. (Citation1987)
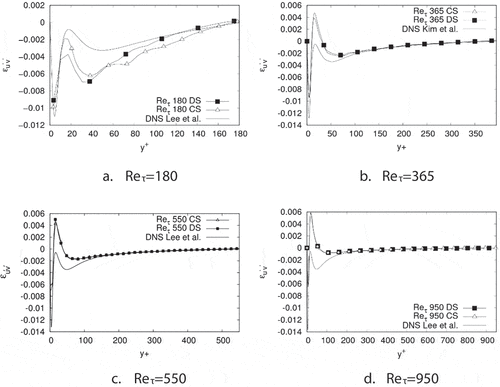
Figure 24. Streamlines for the case with ribs-like protuberances. Coloured bar represents mean streamwise velocity values
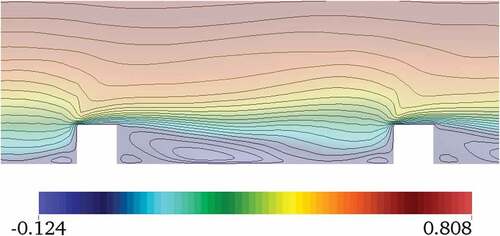
Figure 25. Skin friction coefficient for the case with ribs-like protuberances. Comparison with Dritselis & Vlachos (Citation2011b) and Leonardi et al. (Citation2003). Green pointed line represents the Cf value for a plane channel. Yellow Arrow represents the profile obtained in this paper. CPPE: Channel with ribs-like protuberances analyzed in this paper
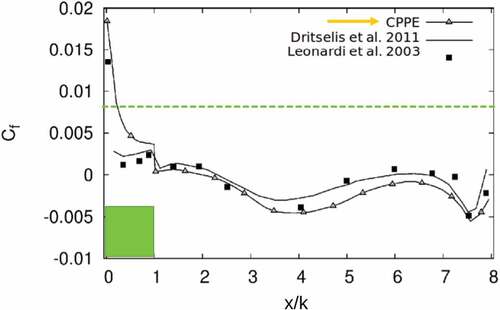
Figure 26. Streamwise mean velocity profiles normalised with Uc for the case with ribs-like protuberances. Uc is the velocity in the central line of the channel. Comparison with Dritselis (Citation2014) and (rlandi et al. (Citation2006). CPPE SP: Profile taken over the perturbation. CPPE EP: Profile taken between perturbations
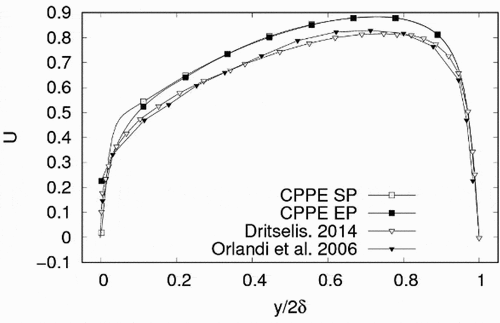
Figure 27. Velocity fluctuations behaviour. Profiles normalised with Ub for the case with ribs-like protuberances. Comparison with Dritselis (Citation2014) and Orlandi et al. (Citation2006). CPPE EP: Profile taken between perturbations
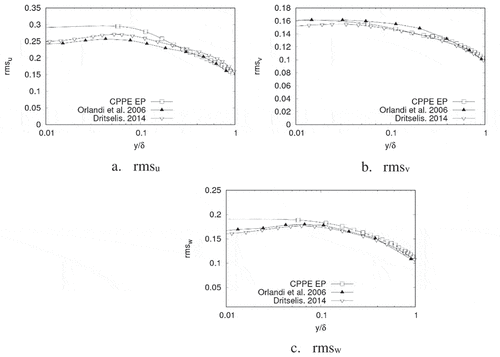
Figure 31. Streamwise mean velocity profile normalised with Uc for the case with cavity-like perturbations. Uc is the velocity in the central line of the channel. Comparison with results obtained with our implementation of the coherent structure SGS model for a plane channel
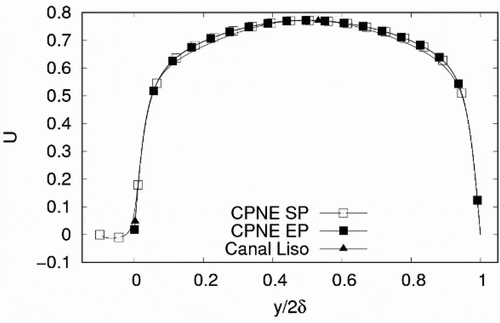
Figure 32. Velocity fluctuations behaviour. Profiles normalised with Ub for the case with cavity-like perturbations. CPNE EP: Profile taken between perturbations; CPNE SP: Profile taken above perturbations. Comparison with results obtained with our implementation of the coherent structure SGS model for a plane channel
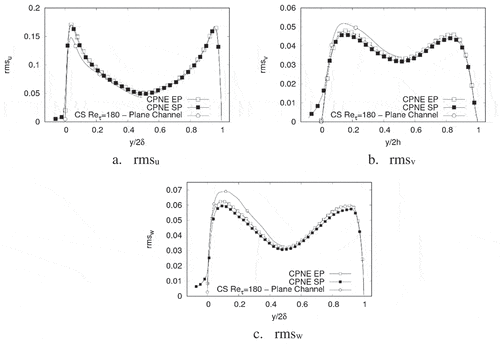