Figures & data
Figure 1. Schematic representation of human behavioral and autonomic adaptations underpinned by the ability to sense humidity and skin wetness. With regards to behavioral responses, variations in ambient humidity and fingertip moisture influence the degree of skin and fingertip wetness; by sensing these changes, humans consciously adapt their thermal behavior and precision grip. With regards to autonomic responses, variations in sweat production and amount of teardrops influence the degree of skin and ocular wetness; by sensing these changes, the body adjust sweat glands’ output and lacrimation via reflex mechanisms.
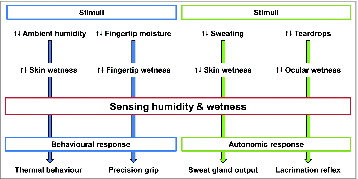
Figure 2. (A) Overview of Bentley's hypothesisCitation12 on wetness perception (A). In his “synthetic experiment,” BentleyCitation12 tested the perception of dipping a sheath-covered finger into a liquid; the results showed that the participants at first refused to believe that the finger was not actually wet. Based on these early observations, Bentley proposed a sensory-blending hypothesis which suggests the blend of light pressure/touch and coldness as responsible for evoking the perception of wetness.Citation12 (B) Biophysical processes (i.e. variation in skin temperature and tactile interactions, with relative activations of skin thermo- and mechanoreceptors) involved in sensing skin wetness. Psychophysical evidence indicates that, in the absence of skin hygroreceptors, humans tend to associate the cold sensations evoked by the drop in skin temperature occurring during the evaporation of moisture from the skin, as a signal of the presence of moisture, and thus wetness, on the skin surface. Also, tactile inputs in the form of light touch and pressure could represent an important source of sensory information for sensing and discriminating skin wetness.
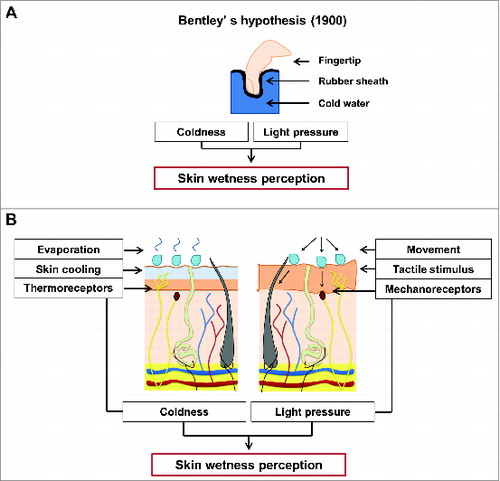
Figure 3. Body maps showing the regional distribution of (A) local skin cooling (°C) caused by the application (10 s) of a cold-dry stimulus previously shown to induce a perception of wetness (i.e. 15°C lower than local skin temperature), (B) absolute mean votes for resulting thermal sensation, (C) and frequency (%) of wetness perception. Regions showing greater skin cooling, colder sensations and more frequent wetness perceptions are represented in darker colors. The rating scale used by the participants to score their absolute thermal is reported next to the respective body map. Two main tendencies are shown. First, the regional differences in thermal and wetness sensation present a similar pattern across the torso (e.g., as opposed to the chest, the lateral and lower back appears more sensitive to cold and wetness). Second, these sensory patterns seem independent from the regional variations in skin cooling (i.e., regions which show greater skin cooling, such as the lateral chest, are not necessarily the ones in which the stimulus was perceived as colder and more often wet). © [The American Physiological Society]. Permission to reuse must be obtained from the rightsholder.Citation62
![Figure 3. Body maps showing the regional distribution of (A) local skin cooling (°C) caused by the application (10 s) of a cold-dry stimulus previously shown to induce a perception of wetness (i.e. 15°C lower than local skin temperature), (B) absolute mean votes for resulting thermal sensation, (C) and frequency (%) of wetness perception. Regions showing greater skin cooling, colder sensations and more frequent wetness perceptions are represented in darker colors. The rating scale used by the participants to score their absolute thermal is reported next to the respective body map. Two main tendencies are shown. First, the regional differences in thermal and wetness sensation present a similar pattern across the torso (e.g., as opposed to the chest, the lateral and lower back appears more sensitive to cold and wetness). Second, these sensory patterns seem independent from the regional variations in skin cooling (i.e., regions which show greater skin cooling, such as the lateral chest, are not necessarily the ones in which the stimulus was perceived as colder and more often wet). © [The American Physiological Society]. Permission to reuse must be obtained from the rightsholder.Citation62](/cms/asset/3b9d4daf-9125-435b-bc05-0a6cd1f33e14/ktmp_a_1008878_f0003_c.jpg)
Figure 4. Summary of sensory mechanisms (i.e., integration of cutaneous temperature and tactile inputs) which seems to underpin humans ability to sense and discriminate between moisture levels on the skin, both when these are the result of a contact with an external stimulus (A–C) as well as when resulting from actively sweating (D).
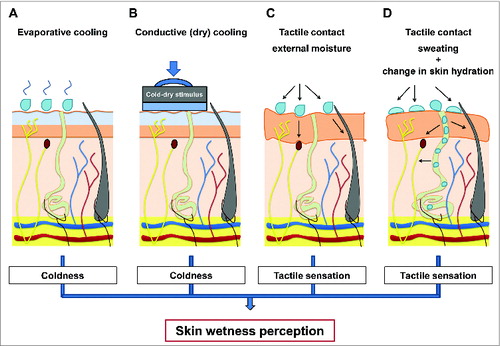
Figure 5. Neurophysiological model of cutaneous wetness sensitivity as developed by Filingeri et al. Mechano Aß, cold Aδ and warm C sensitive nerve fibers and their projections from the skin, through peripheral nerve, spinal cord (via the dorsal-column medial lemniscal pathway and the spinothalamic tract), thalamus and somatosensory cerebral cortex (including the primary and secondary somatosensory cortex cortices SI and SII, the insular cortex and the posterior parietal lobe) are shown. (A and B) Show the neural model of wetness sensitivity (consisting of Aδ and Aß afferents) under normal and under selective reduction in the activity of A-nerve fibers respectively. (C, E and G) Show the pathways for wetness sensitivity during static contact with warm, neutral and cold moisture. (D, F and H) Show the pathways for wetness sensitivity during dynamic contact with moisture. © [The American Physiological Society]. Permission to reuse must be obtained from the rightsholder.Citation11
![Figure 5. Neurophysiological model of cutaneous wetness sensitivity as developed by Filingeri et al. Mechano Aß, cold Aδ and warm C sensitive nerve fibers and their projections from the skin, through peripheral nerve, spinal cord (via the dorsal-column medial lemniscal pathway and the spinothalamic tract), thalamus and somatosensory cerebral cortex (including the primary and secondary somatosensory cortex cortices SI and SII, the insular cortex and the posterior parietal lobe) are shown. (A and B) Show the neural model of wetness sensitivity (consisting of Aδ and Aß afferents) under normal and under selective reduction in the activity of A-nerve fibers respectively. (C, E and G) Show the pathways for wetness sensitivity during static contact with warm, neutral and cold moisture. (D, F and H) Show the pathways for wetness sensitivity during dynamic contact with moisture. © [The American Physiological Society]. Permission to reuse must be obtained from the rightsholder.Citation11](/cms/asset/65f76841-887d-4eff-834a-455111e01b14/ktmp_a_1008878_f0005_c.jpg)
Figure 6. Conceptual model of human hygrosensation. The model comprises biophysical (i.e., thermal and tactile inputs induced by the presence of moisture on the skin), neurophysiological (i.e., central integration of afferents inputs from thermo-sensitive TRP cation channels and nerve fibers and mechano-sensitive DEG/ENaC cation channels and nerve fibers) and psychophysiological mechanisms (i.e., perceptual inference operated by cortical and sub-cortical somatosensory and association areas) which allow humidity and wetness detection in humans. The skin's contact with moisture generates thermal and tactile inputs which are peripherally integrated by specific nervous structures. These inputs evoke thermal and tactile sensations which, in the absence of specific hygroreceptors, are associated to the perception of skin wetness. Repeated exposures to these stimuli (i.e., sensory experience) contribute to generate a neural representation of a typical wet stimulus via learning mechanisms. At this point, only if the learnt combination of stimuli (i.e., coldness and stickiness), as coded by the specific neural afferents (i.e., A-nerve fibers) is presented, wetness will be sensed. In the occurrence of physical wetness on the skin, the bottom-up processes (i.e., combination of thermal and mechanical sensory afferents) as well as the top-down ones (i.e., inference of the potential perception based on the neural representation of a typical wet stimulus) might therefore interact in giving rise (or not) to the perception of wetness.
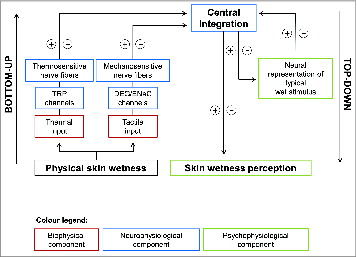