Figures & data
Figure 1. An overview of inflammation and ischemia in obese adipose tissue. Excess fat storage in obese individuals leads to adipose tissue expansion as compared to lean individuals, and the blood supply does not adequately match this tissue expansion. This adipocyte hypertrophy and inadequate blood supply causes adipocyte hypoxia, inflammatory cytokine release (IL-6, TNFα) by adipocytes and M1 macrophages, a reduction in adiponectin release, and impaired insulin action. Low adiponectin promotes the pro-inflammatory profile of macrophages, and adipocytes and macrophages act in a paracrine fashion to further increase cytokine release from neighboring adipose tissue. Insulin resistance in adipocytes causes impaired suppression of lipolysis, and fatty acids are released and deposited in other insulin target tissues such as skeletal muscle and liver. Partially oxidized fatty acids increase inflammation through proteins such as JNK and IKKβ in the liver and skeletal muscle, impairing insulin signaling in these tissues. Impaired insulin action in the liver leads to an increase in glucose release (impaired suppression of glycolysis), and in skeletal muscle leads to decreased glucose uptake (impaired GLUT-4 translocation). The end result is hyperglycemia, hyperlipidemia, meta-inflammation, and insulin resistance. Filled arrows with + signs represent increases in release or uptake, while unfilled arrows with – signs represent decreases in release or uptake.
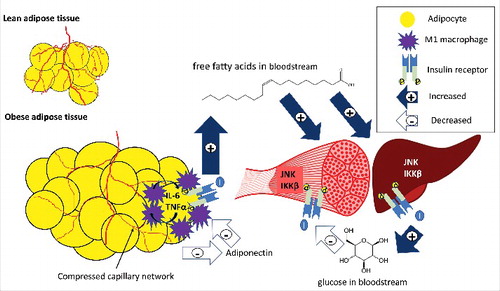
Figure 2. The potential pathways through which chronic heat exposure can reduce inflammation, improve blood flow, and reduce insulin resistance. The increases in shear, HSP90, and HIF1α all offer potential to improve blood flow and reduce adipose tissue hypoxia, through mechanisms such as increased nitric oxide (NO) and vascular endothelial growth factor (VEGF). In addition, HSP70 reduces inflammatory markers in both adipose tissue (nuclear factor kappa-B [NFκB], IL-6, and TNFα) and skeletal muscle (JNK), along with HSP27 (IKKβ). In addition, HSP70 is involved in protein refolding of the insulin receptor. In concert, these mechanisms can reduce inflammation, improve insulin signaling, increase glucose uptake and reduce fatty acid release, and increase adiponectin secretion, improving the metabolic health profile in obesity. While the changes in protein abundance and expression have been experimentally observed in human or animal models (denoted with boxes), some downstream effects have not specifically been examined in response to chronic heat.
![Figure 2. The potential pathways through which chronic heat exposure can reduce inflammation, improve blood flow, and reduce insulin resistance. The increases in shear, HSP90, and HIF1α all offer potential to improve blood flow and reduce adipose tissue hypoxia, through mechanisms such as increased nitric oxide (NO) and vascular endothelial growth factor (VEGF). In addition, HSP70 reduces inflammatory markers in both adipose tissue (nuclear factor kappa-B [NFκB], IL-6, and TNFα) and skeletal muscle (JNK), along with HSP27 (IKKβ). In addition, HSP70 is involved in protein refolding of the insulin receptor. In concert, these mechanisms can reduce inflammation, improve insulin signaling, increase glucose uptake and reduce fatty acid release, and increase adiponectin secretion, improving the metabolic health profile in obesity. While the changes in protein abundance and expression have been experimentally observed in human or animal models (denoted with boxes), some downstream effects have not specifically been examined in response to chronic heat.](/cms/asset/cc0d90ae-e092-41b8-a264-eb5a1d7883f4/ktmp_a_1384089_f0002_oc.jpg)
Figure 3. The potential pathways through which chronic heat exposure can improve cardiovascular health in the heart, macrovasculature, and microvasculature and autonomic activity in the brain. Increases in HSP90 and shear stress with heat therapy act to increase nitric oxide (NO), which can decrease sympathetic outflow, increase vasodilation in the microvasculature, and, along with HIF1α/VEGF, increase angiogenesis in the microvasculature. Increases in angiotensin II type 2 receptors (AT2) and adiponectin in the central nervous system can additionally reduce sympathetic outflow, which can decrease heart rate and peripheral resistance, reducing stress on the cardiovascular system. HSP27 and HSP70 decrease intimal and vascular smooth muscle (VSM) hyperplasia, and HSPs and HIF1α improve ischemic tolerance in the heart, reducing the risk or severity of cardiovascular events. Together, these mechanisms can reduce sympathetic outflow, reduce blood pressure, increase ischemic tolerance, and enhance vascular remodeling to improve the cardiovascular risk profile in obesity. Changes in shear stress, protein abundance and expression have been experimentally observed in human or animal models (indicated with boxes); however; some downstream effects have not specifically been examined in response to chronic heat.
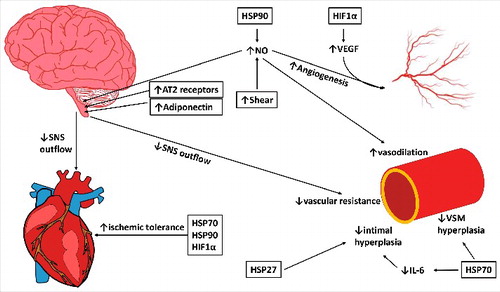