Figures & data
Table 1. Corpus breakdown with basic information of documents in the selected journalsa
Figure 1. ECOC Classification Training (a) Verification that highly relevant articles to either heat stress or autodigestion phenomena seldom discuss both topics in tandem and to similar extents
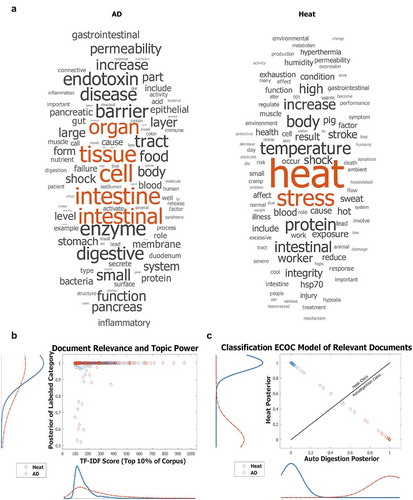
Figure 2. Network maps of LDA Relationships display the topic distributions uncovered from the top 10% of TF-IDF filtered publications. Short-form topic names were manually assigned to LDA-clustered words that represent topics to allow for easier visualization. Edges that connect topic nodes are scaled in size and color by their tandem occurrence in a document. Each document consists of various proportions of all topics. Those topics that account for less than 1/nTopics in an article are removed from consideration for that article and the remaining articles' percentages of accountancy for that document are reweighted. (a) Network map with each node representing a topic. Node size is determined by closeness to centrality. Node color is scaled by betweenness centrality, with darker nodes representing nodes that connect the most topics in any given article within the top 10% percent of TF-IDF filtered papers. Edges connecting two nodes are scaled in color gradient and size by how often the two topics appear in the same report paper. (b) The network map with randomized modularity class detection with a modularity of 0.304 and a resolution of 1. Size of nodes is determined by how often that topic appears in articles of the top 10% percent of TF-IDF filtered papers. Three categories of topics (shown in purple, green, and orange) were uncovered by the network alone, without human intervention or semantic supervision. TF-IDF – Term Frequency Inverse Document Frequency, LDA – Latent Dirichlet Allocation, CNS – Central Nervous System, BMI – Body Mass Index
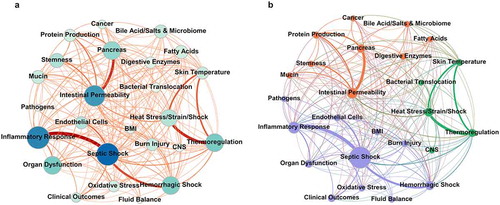
Figure 3. (a) Latent Semantic Analysis (LSA) of words in “Heat” and “Autodigestion (AD)” topics. Three separate word vectorizations were used; two publicly available files downloaded from MathWorks (Fast Text) and Stanford Global Word Vectorization (GloVe) (https://nlp.stanford.edu/projects/glove/), and one embedded from the TF-IDF filtered corpus of scientific articles. Cosine similarity measured the semantic relationships between all pairs of words within and between groups with values ranging from −1 to +1 (directly opposite to nearly identical, respectively). A cosine similarity of 0 indicates orthogonality. (b) Bar graph displaying the use of the “Heat” and “Autodigestion (AD)” terms in journal articles, which have become more frequently used between 2014 and the average of the last 3 years (2019–2021 with 2021 extrapolated due to the timing of this article) in all articles (top), and the number of documents that tandemly employ the two term groups have increased dramatically (normalized to the number of documents published each year). Fold change based on 2014 baseline. (c) Selected topical relationships (edges) from the network diagram in indicate that the relationships between heat stress and intestinal permeability, thermoregulation and digestive enzymes, and intestinal permeability are increasing faster than other intersecting topics examined in the current analysis
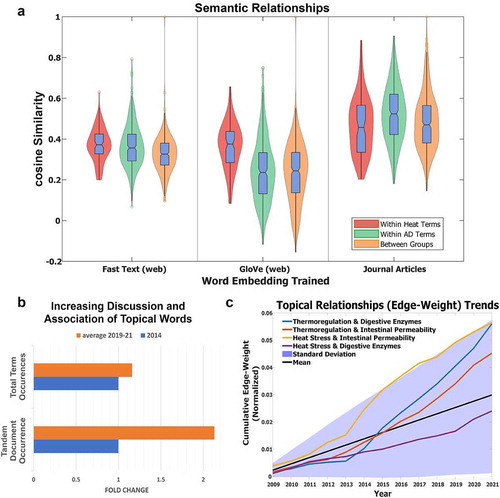
Figure 4. Transmission electron micrographs of small intestinal epithelial cells from one control rat and two rats heated to 42.5°C core temperature. Each image partially depicts two adjacent enterocytes. In heat-stressed rats 1 and 2, there is visible damage to the microvilli compared with the control cells. Bar represents 1 μm. Reprinted with permission from [Citation30], copyright (2002), American Physiological Society
![Figure 4. Transmission electron micrographs of small intestinal epithelial cells from one control rat and two rats heated to 42.5°C core temperature. Each image partially depicts two adjacent enterocytes. In heat-stressed rats 1 and 2, there is visible damage to the microvilli compared with the control cells. Bar represents 1 μm. Reprinted with permission from [Citation30], copyright (2002), American Physiological Society](/cms/asset/bdf5699a-e166-4da4-94d6-8347e5639ea3/ktmp_a_1922261_f0004_b.gif)
Figure 5. Representative light micrographs of H&E (Hematoxylin and Eosin) stained rat everted small intestinal sac tissue over 60 min at core temperatures of 41.5–42°C. Villi appear generally normal (barring slight subepithelial space at villous tips) at 15 min, epithelia begin sloughing from villous tips at 30 min, epithelial lining massively lift from the top and sides of villi at 45 min, and villi were completely denuded at 60 min. Bars represent 100 μm. Between 2 and 4 rats were histologically assessed at each time point. The progression of epithelial damage from the tips of villi to the base of the villi is consistent with findings from other studies. Hall et al. observed that the tips of villi retained significantly more [3H] misonidazole than the middle portion of the villi, which in turn retained significantly more than the base during heat stress Reprinted with permission from [Citation30], copyright (2002), American Physiological Society
![Figure 5. Representative light micrographs of H&E (Hematoxylin and Eosin) stained rat everted small intestinal sac tissue over 60 min at core temperatures of 41.5–42°C. Villi appear generally normal (barring slight subepithelial space at villous tips) at 15 min, epithelia begin sloughing from villous tips at 30 min, epithelial lining massively lift from the top and sides of villi at 45 min, and villi were completely denuded at 60 min. Bars represent 100 μm. Between 2 and 4 rats were histologically assessed at each time point. The progression of epithelial damage from the tips of villi to the base of the villi is consistent with findings from other studies. Hall et al. observed that the tips of villi retained significantly more [3H] misonidazole than the middle portion of the villi, which in turn retained significantly more than the base during heat stress Reprinted with permission from [Citation30], copyright (2002), American Physiological Society](/cms/asset/95590f25-2fa2-4466-a6d5-8f94615b1e22/ktmp_a_1922261_f0005_oc.jpg)
Figure 6. Representative histological images of H&E stained regions of the small intestine in exertional heat stroke (EHS) vs exercise control (EXC) mice. Images were taken 30 min after mice experienced EHS at 37.5°C air temperature or underwent equal intensity and duration of exercise at 25°C air temperature. Reprinted with permission from [Citation59], copyright (2015), American Physiological Society
![Figure 6. Representative histological images of H&E stained regions of the small intestine in exertional heat stroke (EHS) vs exercise control (EXC) mice. Images were taken 30 min after mice experienced EHS at 37.5°C air temperature or underwent equal intensity and duration of exercise at 25°C air temperature. Reprinted with permission from [Citation59], copyright (2015), American Physiological Society](/cms/asset/7114051a-cab4-426a-963c-70bd8d795998/ktmp_a_1922261_f0006_oc.jpg)
Figure 7. Effect of heat stress (HS) on the apoptosis ratio of rat intestinal epithelial cell line (IEC-6) cells. Data are reported as means ± SD, n = 6. *P < 0.01 vs control. Adapted from [Citation25]
![Figure 7. Effect of heat stress (HS) on the apoptosis ratio of rat intestinal epithelial cell line (IEC-6) cells. Data are reported as means ± SD, n = 6. *P < 0.01 vs control. Adapted from [Citation25]](/cms/asset/c80437fa-f62c-48a3-b108-3827568b8fd6/ktmp_a_1922261_f0007_b.gif)
Figure 8. Correlation between core body temperature and intestinal permeability assessed with the urinary lactulose-to-rhamnose (L/R) ratio. The core body temperature and L/R ratio at baseline and at the end of exercise were obtained from the following eight studies: Pals et al. [Citation65], Lambert et al. [Citation66–68], Marchbank et al. [Citation35], Zuhl et al. [Citation69], Zuhl et al. [Citation70] and Davison et al. [Citation42]. See Pires et al. for further detail [Citation44]. Most of the plotted values are expressed as means ± standard error of the mean, while the temperature data in the study of Lambert et al. [Citation67] and the intestinal permeability data in the studies of Lambert et al. [Citation66,Citation67] are expressed as medians. Reprinted with permission from [Citation44], copyright (2016), Springer Nature
![Figure 8. Correlation between core body temperature and intestinal permeability assessed with the urinary lactulose-to-rhamnose (L/R) ratio. The core body temperature and L/R ratio at baseline and at the end of exercise were obtained from the following eight studies: Pals et al. [Citation65], Lambert et al. [Citation66–68], Marchbank et al. [Citation35], Zuhl et al. [Citation69], Zuhl et al. [Citation70] and Davison et al. [Citation42]. See Pires et al. for further detail [Citation44]. Most of the plotted values are expressed as means ± standard error of the mean, while the temperature data in the study of Lambert et al. [Citation67] and the intestinal permeability data in the studies of Lambert et al. [Citation66,Citation67] are expressed as medians. Reprinted with permission from [Citation44], copyright (2016), Springer Nature](/cms/asset/e938c3b4-83ea-48e7-9c18-75392f5c33ec/ktmp_a_1922261_f0008_oc.jpg)
Figure 9. Percentage of human participants showing increased intestinal permeability after exercise according to the core temperature levels attained, calculated by dividing the number of participants reporting augmented intestinal permeability by the total number of participants at each core temperature range as labeled in the bars. Reprinted with permission from [Citation44], copyright (2016), Springer Nature
![Figure 9. Percentage of human participants showing increased intestinal permeability after exercise according to the core temperature levels attained, calculated by dividing the number of participants reporting augmented intestinal permeability by the total number of participants at each core temperature range as labeled in the bars. Reprinted with permission from [Citation44], copyright (2016), Springer Nature](/cms/asset/242f5d83-5b0d-4136-bff7-7d591db052cb/ktmp_a_1922261_f0009_b.gif)
Figure 10. Circulating plasma endotoxin concentrations in trained (TR) and untrained (UT) groups of healthy adult men during exertional heat stress (EHS). Values are means ± SE. From baseline to 38.5°C core temperature (Tc) and exhaustion (Exh), n = 12 TR and 11 UT; at 39.0°C Tc, n = 12 TR and 9 = UT; and at 39.5°C Tc, n = 11 TR. *P < 0.05, UT significantly different from baseline. †P < 0.05, TR significantly different from baseline. ‡P < 0.05, UT significantly different from baseline to 38.5°C Tc. §P < 0.05, between-group significance. Reprinted with permission from [Citation19], copyright (2008), American Physiolgical Society
![Figure 10. Circulating plasma endotoxin concentrations in trained (TR) and untrained (UT) groups of healthy adult men during exertional heat stress (EHS). Values are means ± SE. From baseline to 38.5°C core temperature (Tc) and exhaustion (Exh), n = 12 TR and 11 UT; at 39.0°C Tc, n = 12 TR and 9 = UT; and at 39.5°C Tc, n = 11 TR. *P < 0.05, UT significantly different from baseline. †P < 0.05, TR significantly different from baseline. ‡P < 0.05, UT significantly different from baseline to 38.5°C Tc. §P < 0.05, between-group significance. Reprinted with permission from [Citation19], copyright (2008), American Physiolgical Society](/cms/asset/53d41c95-1c1c-44ab-b84a-33a09bbd0550/ktmp_a_1922261_f0010_b.gif)
Figure 11. Correlation of lipopolysaccharide (LPS), tumor necrosis factor (TNF), and interleukin 1 (IL-1) with rectal temperature during heat stroke. Data points represent measurements taken from 17 adult patients with mean rectal temperatures between 40.6 and 43.3°C. Reprinted with permission from [Citation9], copyright (1991), American Physiological Society
![Figure 11. Correlation of lipopolysaccharide (LPS), tumor necrosis factor (TNF), and interleukin 1 (IL-1) with rectal temperature during heat stroke. Data points represent measurements taken from 17 adult patients with mean rectal temperatures between 40.6 and 43.3°C. Reprinted with permission from [Citation9], copyright (1991), American Physiological Society](/cms/asset/14eba0de-66a0-4949-9a97-c4c403df4be4/ktmp_a_1922261_f0011_b.gif)
Figure 12. Blood glucose levels in mice taken at various time points into recovery post-exertional heat stroke (EHS) or -exercise control (EXC) compared with a naive control group (NC). A: 0.5 h post-EHS or -EXC. B: 3 h post-EHS or -EXC. C: 24 h post-EHS or -EXC. D: 4 days post-EHS; glucose tests were only available for EHS groups receiving 30% and 90% relative humidity at 37.5°C ambient temperature. Data are medians ± 25–75% ranges. *P < 0.05, **P < 0.01. Reprinted with permission from [Citation59], copyright (2015), American Physiological Society
![Figure 12. Blood glucose levels in mice taken at various time points into recovery post-exertional heat stroke (EHS) or -exercise control (EXC) compared with a naive control group (NC). A: 0.5 h post-EHS or -EXC. B: 3 h post-EHS or -EXC. C: 24 h post-EHS or -EXC. D: 4 days post-EHS; glucose tests were only available for EHS groups receiving 30% and 90% relative humidity at 37.5°C ambient temperature. Data are medians ± 25–75% ranges. *P < 0.05, **P < 0.01. Reprinted with permission from [Citation59], copyright (2015), American Physiological Society](/cms/asset/d6c6ac01-a079-4c57-8707-5d2cd167d181/ktmp_a_1922261_f0012_b.gif)
Figure 13. Effects of heat stress and protease-activated receptor 1 (PAR1) inhibitor RWJ58,259 on mouse intestinal permeability as assessed with the urinary lactulose-to-rhamnose (L/R) ratio. The heat stressed (HS) and HS + RWJ58,259 mice had core temperatures of 41°C and recovered at room temperature for 24 h. In the HS + RWJ-58259 group, mice were injected with RWJ-58259 (10 mg/kg) via a tail vein 30 min before heat stress. In the Control group, the mice were injected with a vehicle solution and left at room temperature for the same amount of time. Data are mean ± SD. *P < 0.05 vs Control, #P < 0.05 vs HS, n = 6–8. Reprinted with permission from [Citation41]
![Figure 13. Effects of heat stress and protease-activated receptor 1 (PAR1) inhibitor RWJ58,259 on mouse intestinal permeability as assessed with the urinary lactulose-to-rhamnose (L/R) ratio. The heat stressed (HS) and HS + RWJ58,259 mice had core temperatures of 41°C and recovered at room temperature for 24 h. In the HS + RWJ-58259 group, mice were injected with RWJ-58259 (10 mg/kg) via a tail vein 30 min before heat stress. In the Control group, the mice were injected with a vehicle solution and left at room temperature for the same amount of time. Data are mean ± SD. *P < 0.05 vs Control, #P < 0.05 vs HS, n = 6–8. Reprinted with permission from [Citation41]](/cms/asset/ec057407-66ae-404d-9eba-76f8145c6206/ktmp_a_1922261_f0013_b.gif)
Figure 14. Summary of a hypothesis that links heat strain, elevated intestinal permeability to leak of digestive enzymes from the lumen of the intestine into the systemic circulation. During healthy digestion (left) digestive enzymes are compartmentalized within the small intestine. After heat stress (left) they leak into the systemic circulation, cause cell and tissue failure by cleavage of plasma proteins and membrane receptors, heat illness, an inflammatory response, shock and organ dysfunctions. Drawings courtesy of Peter J. Schmid-Schoenbein.
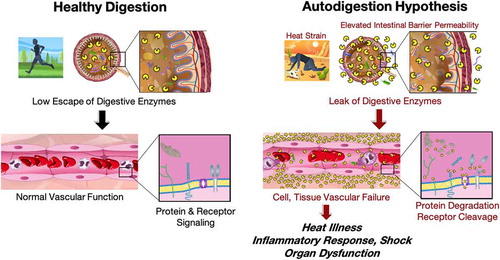
Supplemental Material
Download PDF (398 KB)Data availability statement
Please contact Anthony A. Fung ([email protected]) for the corpus code, which is comprised of commercial MATLAB toolboxes, as well as network diagrams made in Gephi.