Figures & data
Fig. 7. Tube shapes: a. Round; b. Ellipse; c. Eye; d. Airfoil leading edge; e. Airfoil trailing edge.

Fig. 8. Local thermal-hydraulic characteristics for round tube at constant Reynolds: a. hydraulic boundary layer; b. temperature gradient at the wall; c. tangential velocity gradient at the wall.
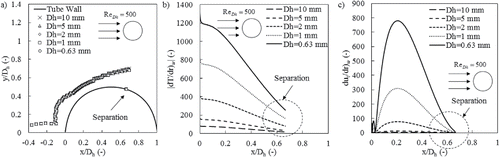
Fig. 9. Local thermal-hydraulic characteristics for round tube at constant velocity: a. hydraulic boundary layer; b. temperature gradient at the wall; c. tangential velocity gradient at the wall.
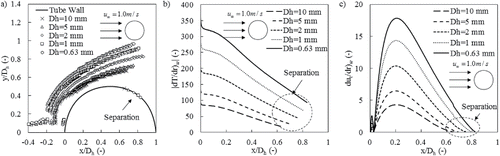
Table 1. NTHX-001 Numerical results compared to the baseline MCHX.
Table 2. Design space.