Figures & data
Figure 1. General structure of a canonical HSP70. The NBD, also known as the ATPase domain, is connected by a highly conserved linker to the SBD. The SBD, also known as the peptide binding domain, is characterized by an alpha-helical lid (red) which covers or exposes the binding site. Structure generated using PRIMO server (Hatherley et al. Citation2016).
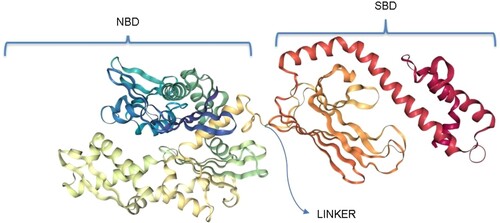
Figure 2. The EEVD/N motif of the HSP70s across species reported with their corresponding Uniprot accession codes. PfHSP70-X has a variant motif, EEVN, from the common EEVD. PfHSP70-1 and PfHSP70-1 EEVD/N motif is preceded by a valine residue and not isoleucine like every other HSP70.
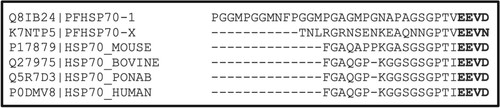
Figure 3. The top box shows the GGMP motifs unique to PfHSP70-1. The bottom box shows the EKEK motif unique only to PfHSP70-z. The proteins were identified using Uniprot accession numbers.
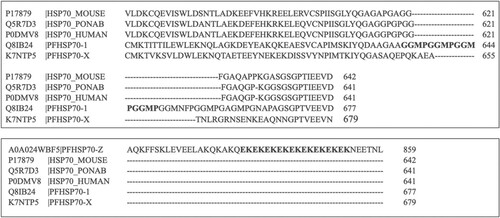
Figure 4. This illustrates how PfHSP70-1 ATPase cycle undergoes, ATP/ADP interchange and the interaction with PfHSP40. The green figures represent ATP-bound state of PfHSP70-1 and the blue figure, the ADP-bound state.
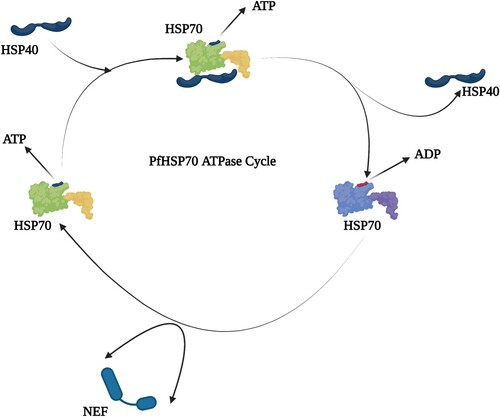
Figure 5. The structure of the complex of HSP70 bound to J-domain (HSP40) with all the domains shown as labelled. The J-domain facilitates cross talks with HSP70 through binding to both the SBD and NBD while closely packed against the linker of the HSP70 (PDB ID:5NRO) (Kityk et al. Citation2018).
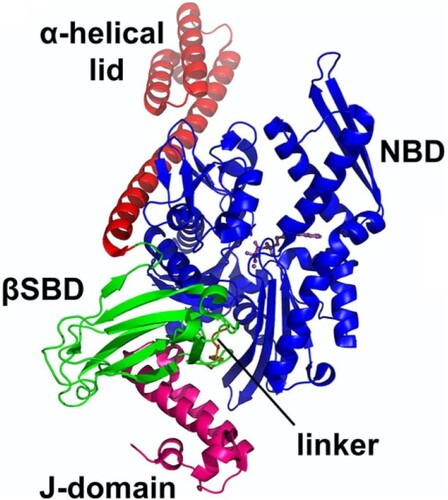
Figure 6. The general structure of the J-domain of an HSP40 (pdb: hdj1): Four helices numbered I–IV. The chain terminals are labelled C and N, respectively. The highly conserved His31 (red), Pro32 (blue), Asp33 (yellow) shown as HPD between Helices II–III.
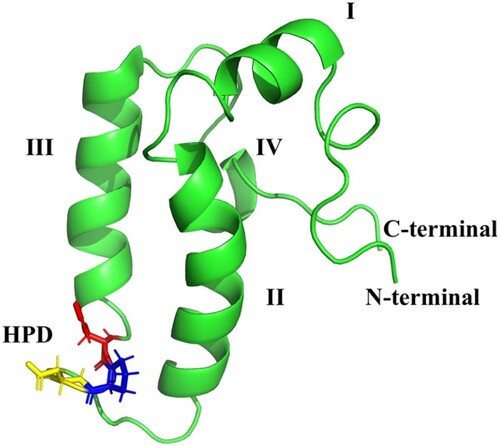
Figure 7. The classification of HSP40s according to domain organization and suggested functions of the domains. Type I possesses the J-domain, the glycine/phenylalanine (G/F) rich region, and zinc binding cysteine rich region which consists of CXXCXGXG repeats and a less conserved carboxyl-terminal domain (120–170 amino acids). Type II possesses the J-domain, the G/F rich region, and the carboxyl-terminal. Type I and II are believed to have similar functions and to bind to non-native substrates. Type III only possesses the J-domain and does not bind to non-native substrates. It has been suggested that it cannot function as an individual chaperone, and the J-domain may be located anywhere in the molecule. Type IV, first identified by Botha et al. (Citation2007) possesses the J-domain with variations in the HPD motif.
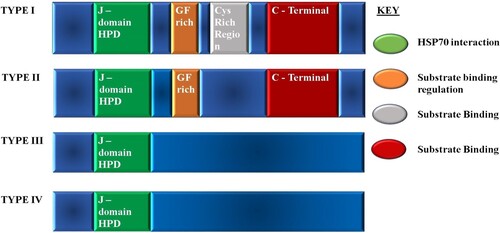
Figure 8. This figure summarizes the concert interplay of the chaperone machinery of P. falciparum. The chaperone’s main functional partners are known to be the PfHSP40s which act as substrate scanners and PfHSP90s for functional maturation via the PfHoP.
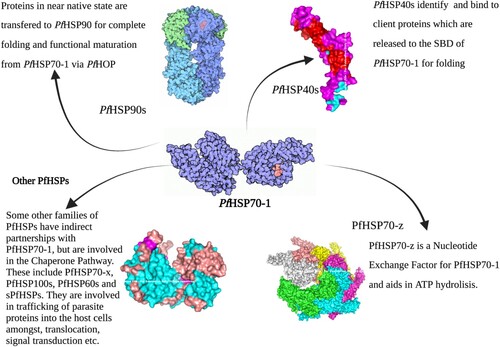
Figure 9. 15-DSG stalled protein synthesis in P. falciparum through PfHsp70 sequestration and phosphorylation of eukaryotic initiation factor (eIF2a) (Ramya et al. Citation2006; Fewell et al. Citation2004; Ramya et al. Citation2007).
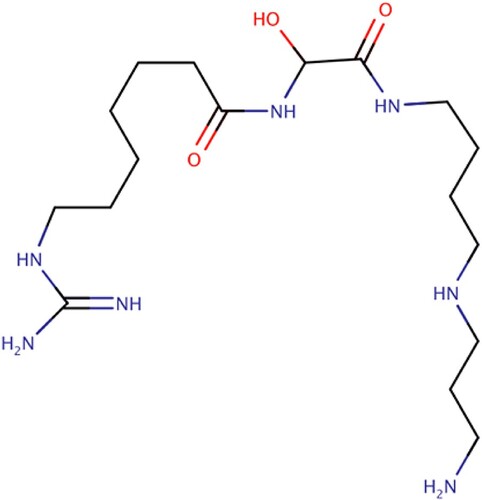
Figure 10. Pyrimidinones with in vitro activity against the ATPase activity of PfHsp70-1. These pyrimidinones are known to enhance the ATP hydrolysis of HSP70 as such have potential to affect the ability of PfHsp70-1 to act as a chaperone (Chiang et al. Citation2009).
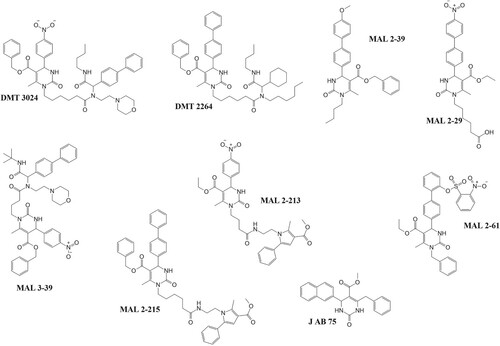
Figure 11. Chemical structures of PfHsp70-1 inhibitors: These compounds (with the exception of SANCs) inhibit the growth of the parasite and also suppress PfHsp70-1’s aggregation suppression function. SANC compounds induce allosteric conformational dynamics affecting nucleotide/substrate release events (Cockburn et al. Citation2011; Zininga et al. Citation2017a, Citation2017b, Citation2017c; Amusengeri et al. Citation2019).
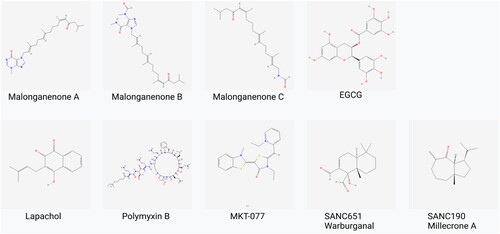
Data availability statement
Data sharing not applicable to this article as no datasets were generated or analysed during the current study.