Figures & data
Figure 3. Basic principle of the use of enzyme artificial cells for implantation in inborn errors of metabolism (e.g., acatelasemia) and cancer (lymphosarcoma).
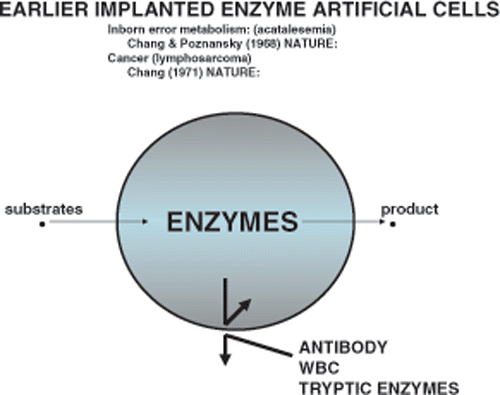
Figure 4. Our recent approach of giving artificial cells orally to avoid the need for implantation. This way, each artificial cell as it travels through the intestine, acts as a microscopic dialyzer. By placing enzyme or other bioreactants inside the artificial cells, they can act as combined dialyzer–bioreactors on their passage through the intestine. After carrying out their function, they are excreted in the stool. Thus there is no accumulation in the body.
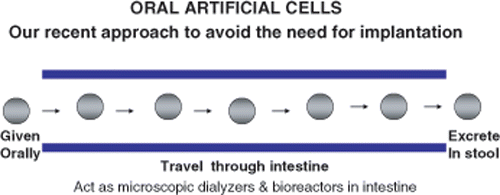
Figure 5. Oral artificial cells containing phenylalanine ammonia lyase for the removal of systemic phenylalanine in the most common inborn error of metabolism, phenylketonuria.
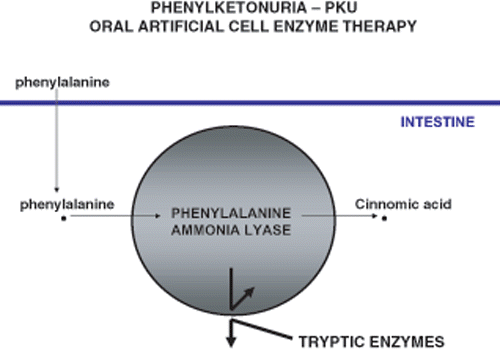
Figure 6. Our new finding that there is an extensive recirculation of amino acids between the body and the intestine. Pancreatic, gastric, intestinal, and other secretions from the body contains a large amount of protein, peptides, and other sources of amino acids. These are digested in the intestine into amino acids that are reabsorbed into the body. Artificial cells containing one enzyme to break down one amino acid can thus break the recirculation for this particular amino acid resulting in decreasing its concentration in the body.
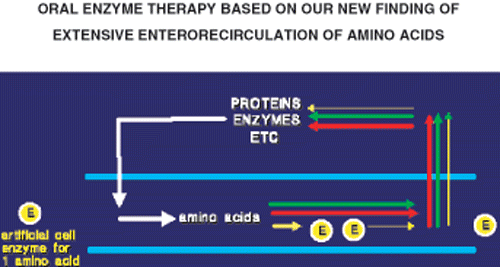
Figure 7. Oral artificial cells containing tyrosinase can selectively lower the systemic tyrosine level.
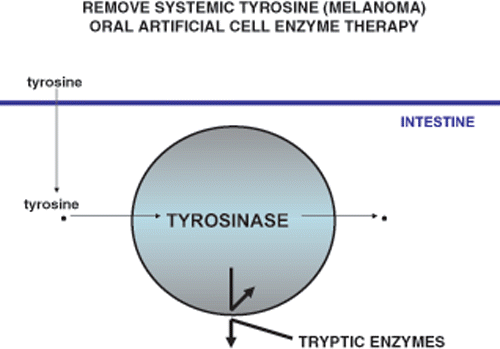
Figure 8. Unlike amino acid, hypoxanthine is very lipid soluble and can thus equilibrate rapidly with the intestinal content. Artificial cells containing xanthine oxidase can therefore lower the elevated systemic hypoxanthine level in a patient with Lesch-Nyhan disease.
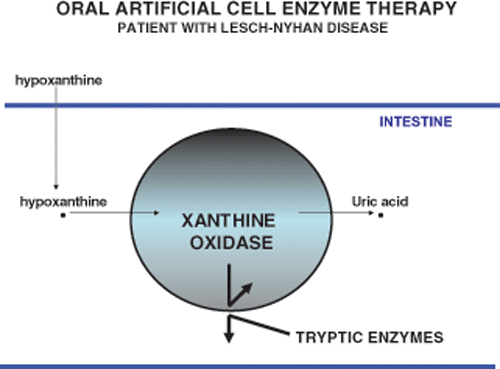
Figure 9. Basic principle of artificial cells for cell encapsulation. The encapsulated cells are retained inside the microcapsules and thus isolated from the external environment. This prevents immunological rejection of the encapsulated cells. However, the membrane can be made permeable to oxygen and nutrients needed by the cell. Also, the products of the cells like insulin, peptide, and other materials can leave the microcapsules.

Figure 10. Co-encapsulation of cells with stem cells to increase the viability of the encapsulated cells.
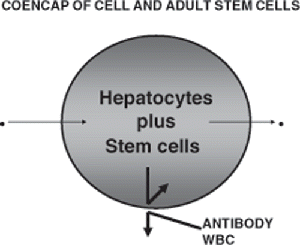
Figure 11. Experiment showing that co-encapsulation with stem cells increases the viability of hepatocytes after implantation.
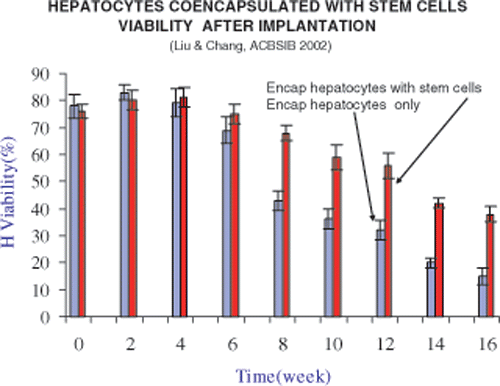
Figure 12. Basic principle of bioencapsulation of genetically engineered cells is the same as for cell encapsulation.
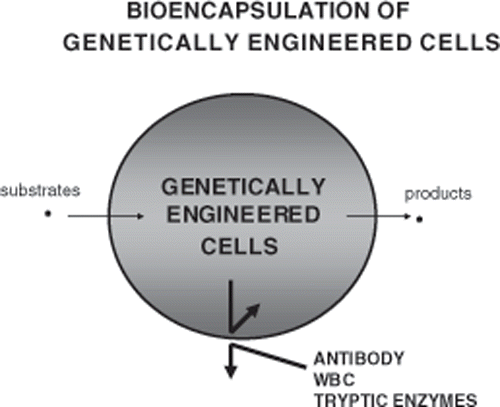
Figure 13. Molecular dimension red blood cell substitutes in the form of polyhemoglobin. This is formed by the intermolecular crosslinking of hemoglobin into a soluble complex. In this form, they are retained in the circulation.
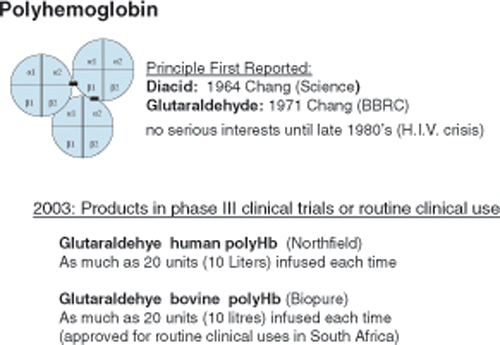
Figure 14. Comparison of polyhemoglobin with donor red blood cells. Polyhemoglobin has many advantages over red blood cells and is useful for use during surgery. However, it cannot be used in a number of other clinical conditions. This is because unlike red blood cells (RBC), polyhemoglobin is only an oxygen carrier. It does not have RBC enzymes needed for many functions including the removal of oxygen radicals. Furthermore, its circulation time is much shorter than that of RBC.
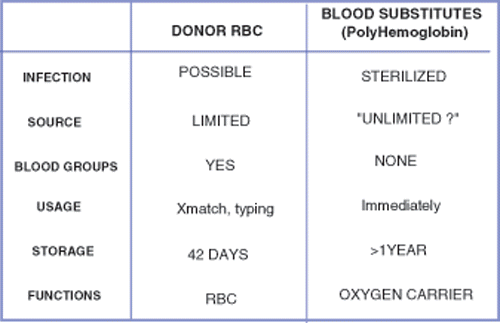
Figure 15. Crosslinking of hemoglobin with two RBC enzymes to form polyhemoglobin-catalase-superoxide dismutase (PolyHb-CAT-SOD). Unlike polyhemoglobin, this has RBC enzymes that can remove oxygen radicals.
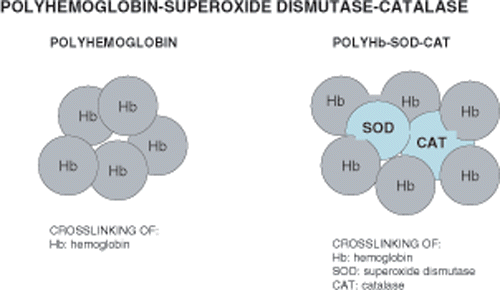
Figure 16. In conditions like severe sustained hemorrhagic shock, stroke, myocardial infarction and organ transplantation, reperfusion with polyhemoglobin can sometimes result in oxygen radicals that causes tissue injury. PolyHb-CAT-SOD can supply oxygen and at the same time significantly lower any oxygen radicals formed.

Figure 17. This is a rat model of acute global cerebral ischemia followed by reperfusion with different oxygen carrying solutions. Unlike polyhemoglobin, polyHb-CAT-SOD does not cause brain edema when used in this situation.
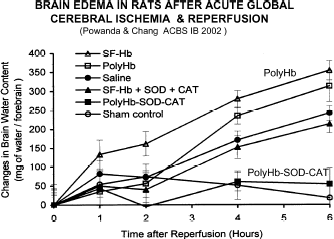
Figure 18. Nanodimension artificial red blood cells with polyethylene-glyco-polylactide membrane. In addition to hemoglobin, this contains the same enzymes that are normally present in red blood cells. Thus, it has the complete function of the red blood cells.
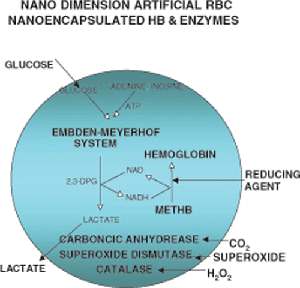
Figure 19. Nanodimension artificial red blood cells with polyethylene-glyco-polylactide membrane. The circulation time is double that of polyhemoglobin. The circulation half time of polyhemoglobin in human is about 24 hours. This means that the nanodimension artificial RBC may have a circulation time of about 48 h in human.
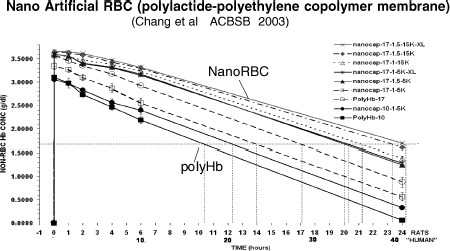