Figures & data
Figure 1. Oxidative stress is an imbalance between reactive oxygen species (ROS) and antioxidants. (A) Under normal conditions there are sufficient antioxidants in the lung to overcome endogenous ROS. (B) During pathologic states there is an increase in ROS that may be balanced by increase in antioxidants. (C) When either ROS production is excessive or antioxidants are inadequate, the balance is tipped toward oxidative stress and injury from ROS may occur. (Full color version available online.)
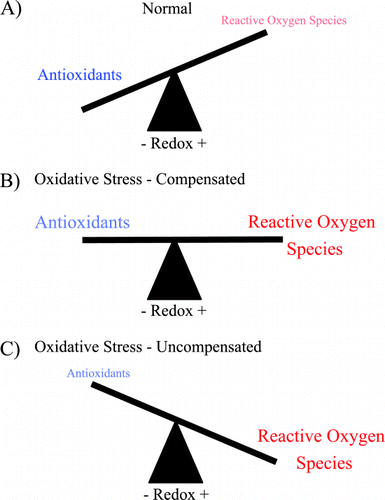
Figure 2. Derivation of reactive oxygen species. Sequential electron (e−) addition to oxygen results in the formation of reactive oxygen species (red): superoxide (O2‐), hydrogen peroxide (H202) and hydroxyl radical (OH). Superoxide can combine with nitric oxide (NO) to form peroxynitrite (ONOO‐). Both O2− and OH− can initiate lipid peroxidation to form lipid peroxides (ROO) that can propagate free radical chain reactions. (Full color version available online.)
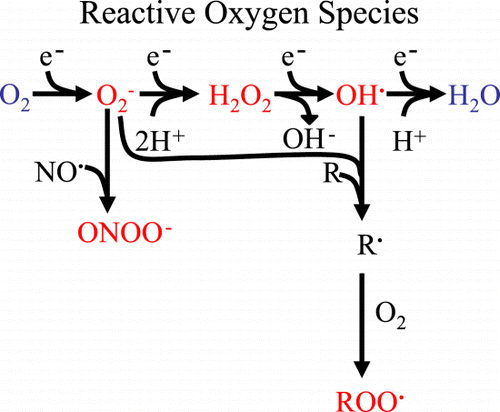
Figure 3. Oxidants in cigarette smoke. Nitric oxide (NO), nitrogen dioxides (NO2), carbon monoxide (CO), ammonia (NH3), cyanide (HCN), hydrogen peroxide (H2O2), superoxide (O2−), and peroxynitrite (ONOO‐). (Full color version available online.)
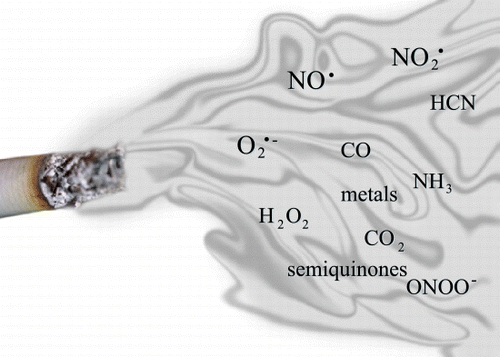
Table 1. Low Molecular Weight Antioxidants in Normal Subjects
Figure 4. Pulmonary distribution of extracellular superoxide dismutase. Histochemical localization of the superoxide dismutases in human lung using rabbit anti‐human extracellular superoxide dismutase extracellular superoxide dismutase (ECSOD) antibodies. ECSOD labeling of a small airway (A) and small pulmonary vessel (B). Strong labeling is seen in the airway and vessel walls (arrows). (A) Reprinted by permission from Ref. Citation[[206]] and (B) reprinted by permission from Ref. Citation[[207]]. (Full color version available online.)
![Figure 4. Pulmonary distribution of extracellular superoxide dismutase. Histochemical localization of the superoxide dismutases in human lung using rabbit anti‐human extracellular superoxide dismutase extracellular superoxide dismutase (ECSOD) antibodies. ECSOD labeling of a small airway (A) and small pulmonary vessel (B). Strong labeling is seen in the airway and vessel walls (arrows). (A) Reprinted by permission from Ref. Citation[[206]] and (B) reprinted by permission from Ref. Citation[[207]]. (Full color version available online.)](/cms/asset/ffaec02e-0308-4c60-b3c6-d721728c3c4a/icop_a_27031_uf0004_b.gif)
Table 2. Free Radicals and Their Footprints in COPD
Figure 5. Treatment with an SOD mimetic (M40419) attenuates anti‐VEGF‐(SU5416) mediated emphysema. (a) SU5416‐treated lungs show increased size of alveolar structures. (b) SU5416 + M40419‐treatment protects against alveolar loss and these animals show normal alveolar size and architecture (bar = 100 µm). Adapted with permission from Ref. Citation[[150]]. (Full color version available online.)
![Figure 5. Treatment with an SOD mimetic (M40419) attenuates anti‐VEGF‐(SU5416) mediated emphysema. (a) SU5416‐treated lungs show increased size of alveolar structures. (b) SU5416 + M40419‐treatment protects against alveolar loss and these animals show normal alveolar size and architecture (bar = 100 µm). Adapted with permission from Ref. Citation[[150]]. (Full color version available online.)](/cms/asset/c7f88757-a772-4914-ab04-b3095ab76835/icop_a_27031_uf0005_b.gif)
Figure 6. Stimulation of normal alveolar macrophages activates nuclear factor‐κB (NF‐κB) and other transcription factors to switch on histone acetyltransferase leading to histone acetylation and transcription of genes encoding inflammatory proteins, such as tumor necrosis factor‐α (TNF‐α), interleukin‐8 (IL‐8) and matrix metalloproteinase‐9 (MMP‐9). Corticosteroids reverse this by binding to glucocorticoid receptors (GR) and recruiting histone deacetylase‐2 (HDAC2). This reverses the histone acetylation induced by NF‐κB and switches off the activated inflammatory genes. In COPD patients cigarette smoke activates macrophages, as in normal subjects, but oxidative stress (perhaps acting through the formation of peroxynitrite) impairs the activity of HDAC2. This both amplifies the inflammatory response to NF‐κB activation, and reduces the anti‐inflammatory effect of corticosteroids as HDAC2 is now unable to reverse histone acetylation. (Full color version available online.)
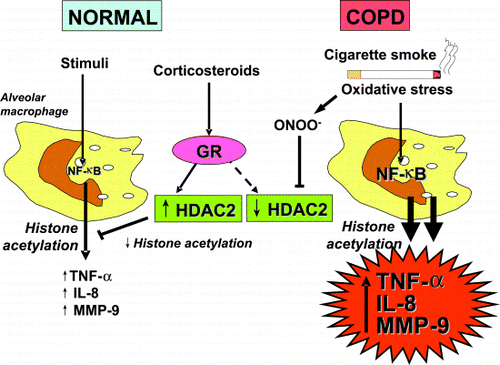
Figure 7. Low levels of plasma ascorbate increase the risk of COPD independently of smoking history. The relative risks of having COPD in light (white bar), medium (black bar) and heavy (cross hatched bar) smokers compared to never smokers are shown for each quintile of plasma ascorbate concentration. The risk of COPD was highest in the patients with the very low quintile of plasma concentration (3–37 µM), compared to low (28–49 µM), median (50–58 µM), high (59–68 µM), or very high quintiles (69–174 µM). Adapted from Ref. (176).
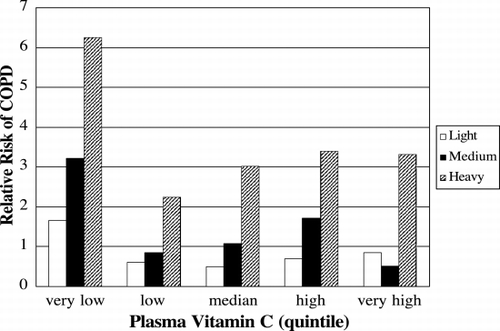
Table 3. Randomized Controlled Trials Using N‐Acetylcysteine to Treat COPD Patients
Figure 9. Effects of tobacco smoke on airways. Catalytic antioxidants attenuate squamous metaplasia from tobacco smoke. (A) Epithelial cell changes in airways from tobacco smoke‐exposed and filtered air (control) rats (epithelium is indicated between arrows). Lung sections were stained with hematoxylin and eosin for general morphology. Airway epithelium from filtered air control rats do not show the striking squamous cell metaplasia with prominent filamentous‐like cytoplasmic extensions (*) observed in the airways from rats exposed to tobacco smoke for 8 weeks. (B) Squamous metaplasia in rat lungs with or without a superoxide dismutase mimetic. The percent of airway surface expressing the type of squamous metaplasia illustrated in is shown. Data are presented as mean ± SE (n = 5−6). *p < 0.05 for comparison to the tobacco smoke alone group. Adapted from Ref. Citation[[57]]. (Full color version available online.)
![Figure 9. Effects of tobacco smoke on airways. Catalytic antioxidants attenuate squamous metaplasia from tobacco smoke. (A) Epithelial cell changes in airways from tobacco smoke‐exposed and filtered air (control) rats (epithelium is indicated between arrows). Lung sections were stained with hematoxylin and eosin for general morphology. Airway epithelium from filtered air control rats do not show the striking squamous cell metaplasia with prominent filamentous‐like cytoplasmic extensions (*) observed in the airways from rats exposed to tobacco smoke for 8 weeks. (B) Squamous metaplasia in rat lungs with or without a superoxide dismutase mimetic. The percent of airway surface expressing the type of squamous metaplasia illustrated in Fig. 9A is shown. Data are presented as mean ± SE (n = 5−6). *p < 0.05 for comparison to the tobacco smoke alone group. Adapted from Ref. Citation[[57]]. (Full color version available online.)](/cms/asset/4cb06350-f0ec-4302-a976-132774203098/icop_a_27031_uf0009_b.gif)