Figures & data
Figure 1 Neointima formation following coronary stenting is reduced with Ni-free stainless steel stents. (a) Representative x-ray photographs of SUS316L stainless and Ni-free stainless stents within isolated porcine coronary arteries 28 days after implantation. (b)–(g) Coronary stents made of SUS316L or Ni-free stainless steel were implanted in the left anterior descending and circumflex arteries of pigs. The stented regions were then analyzed using radiography and intravascular ultrasound imaging, after which the stented arteries were harvested. In (b), representative angiographic views of the porcine left circumflex arteries 28 days after stent implantation are shown. All angulation was approximately right anterior oblique 50. Arrowheads indicate the ends of the stent-implanted sites in the left circumflex arteries. (c) Per cent in-stent restenosis evaluated using angiography; n = 20 and 17 in the SUS315L and Ni-free stent groups, respectively. ∗P < 0.05. (d) Representative sections of stented arteries stained with hematoxylin/eosin. L: lumen, N: neointima, M: media, bars: 500 μm. For morphometric analysis, luminal areas were defined as the blank areas within arteries. The smooth muscle layer, including the neointima and media, was defined as the inner side (high cell density) area of the vascular wall, while the adventitia was defined as the outer side (low cell density) area. The borders of the neointima (inner side of the smooth muscle layer) and media (outer side of the smooth muscle layer) were defined as the boundaries between two different cell alignments. In addition, in-stent neointima formation was evaluated at three positions within each stent (proximal, middle or distal), and the data from the position that showed the largest intimal area/medial area ratio were selected as representative for that stent. Results of histological analyses of the intimal area (e), intimal area/medial area ratio (f) and lumen area (g); n = 20 and 17 in the SUS316L and Ni-free stent groups, respectively. ∗P < 0.05.
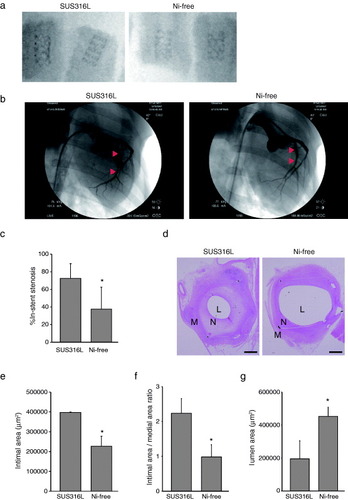
Figure 2 Reduced inflammatory cell infiltration and fibrotic changes following Ni-free stent implantation. Photomicrographs of cross-sections of porcine coronary arteries 28 days after implantation of SUS316L or Ni-free stainless stents. Hematoxylin/eosin staining (a, b) and Masson's trichrome staining (c). Ni-free stainless stent-implanted arteries showed significantly less inflammatory cell infiltration, as indicated by the smaller number of hematoxylin-positive mononuclear cells (a, b) and smaller areas of collagen deposition (blue signal), as compared to SUS316L stainless stent-implanted vessel segments (c). L: lumen, N: neointima, M: media, ∗: stent struts, scale bars: 1 mm (a, c) and 100 μm (b).
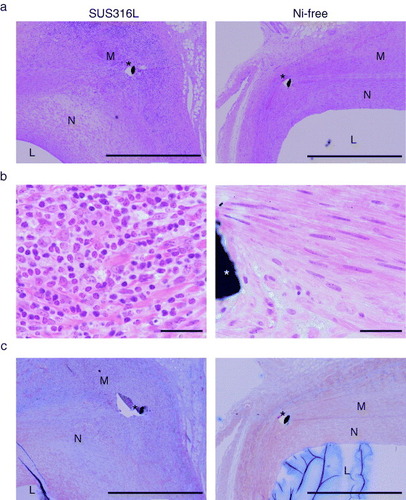
Figure 3 Ni2+ activates HIF-1α in vascular SMCs. (a) Dissolution of Ni2+ from SUS316L and Ni-free stainless into saline solution, ∗P < 0.05. (b) Western blots of HIF-1α in cultured vascular SMCs supplemented with Ni2+ for 6 h under normoxic conditions. Ni2+ stabilized HIF-1α in cultured vascular SMCs under normoxic conditions. (c) Hypoxia modestly induced HIF-1α target genes (Vegfa, Adm2 and Pdgfb) in cultured SMCs; n = 3 in each group, ∗P < 0.05 versus control, ∗∗P < 0.05 versus 0.5 mM Ni under the same O2 conditions. #P < 0.05 versus the same Ni concentration group in 20% O2.
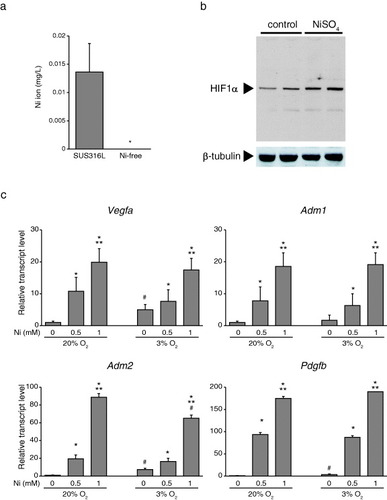
Figure 4 Schematic of HIF-1α target gene activation in vascular smooth muscle cells by Ni2+ from a SUS316L stent. Under normoxic conditions within smooth muscle cells, HIF1-α is post-translationally hydroxylated by PHDs (prolyl hydroxylase domain-containing enzymes), and this hydroxylation leads to pVHL (von-Hippel–Lindau protein)-dependent ubiquitination and degradation of HIF-1α in the cytoplasm. Fe2+ is required for the reaction catalyzed by PHDs. Under hypoxic conditions, the degradation process is blocked, enabling HIF1-α to enter the nucleus and induce expression of its target genes, which include Vegfa, Pdgfb, Adm1 and Adm2. Even under normoxic conditions, Ni2+ may displace the Fe2+ from its binding site on PHDs, leading to HIF1-α activation.
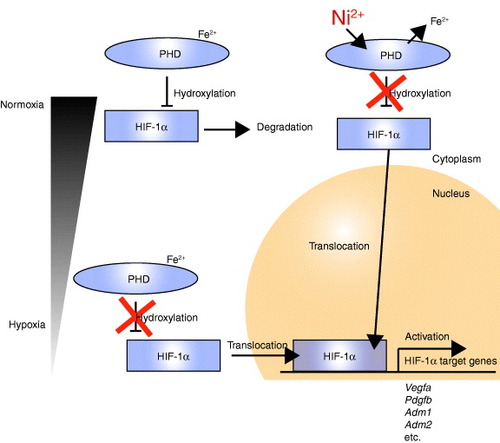
Figure 5 Reduced Hif-1α activation in vascular walls receiving Ni-free stainless stents. (a) Immunohistochemical detection of HIF-1α within stented arterial walls 28 days after stent implantation. N: neointima, M: media, A: arterial lumens, S: stent struts. HIF-1α is shown in yellow and SM α-actin, a SMC marker, is shown in red. Scale bars: 50 μm. (b) Expression levels of the HIF-1α target genes Vegfa and Pdgfa and the inflammatory cytokine Tnf in the walls stented arteries. ∗P < 0.05 versus control, #P < 0.05 versus SUS316L stent.
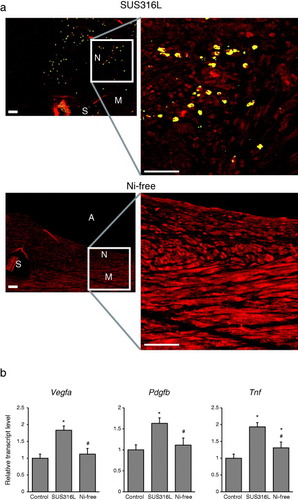