Figures & data
TABLE 1 S. cerevisiae proteins containing at least two Fpk1 phospho-acceptor site motifs
FIG 1 Absence of Fpk1 phosphorylation impairs PtdEth flipping by Dnf1. (A) Strains GFY1770 (DNF1 dnf2Δ dnf3Δ), GFY1773 (DNF1-GFP dnf2Δ dnf3Δ), GFY1772 (DNF1 6A dnf2Δ dnf3Δ), GFY1775 (DNF1 6A -GFP dnf2Δ dnf3Δ), and GFY1728 (dnf1Δ dnf2Δ dnf3Δ) were plated as a lawn on yeast extract-peptone-dextrose (YPD) plates, and 10 μl of stock solution of duramycin (either 8 mM or 4 mM) was spotted onto sterile filter paper disks which were immediately placed onto the lawn. Plates were scanned after incubation at 30°C for 2 days. (B) Extracts from GFY1728, GFY1773, and GFY1775 cells were resolved by SDS-PAGE and analyzed by immunoblotting with anti-GFP antibodies. (C) The same cells as in panel B, costained with CellMask Orange to highlight the plasma membrane, were viewed by fluorescence microscopy as described in Materials and Methods.
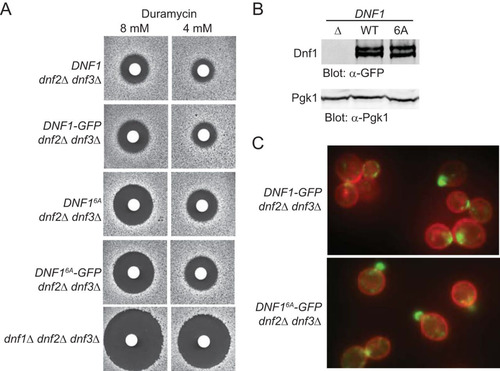
FIG 2 Fpk1 phosphorylates Akl1 at S960 and S1072. (A) Schematic representation of the Akl1, Ark1, and Prk1 protein kinases. The catalytic domain (red rectangle) is near the amino terminus. Akl1 has the longest C-terminal segment, and only Akl1 contains consensus Fpk1 phospho-acceptor site motifs, both near its C terminus. (B) GST-Fpk1 (pAX15, WT) or catalytically inactive (kinase-dead [KD]) mutant GST-Fpk1(D621A) (pJY10) were purified from E. coli, incubated with [γ-32P]ATP and either GST-Akl1(767-1108) (pFR290), GST-Akl1(767-1108; S960A) (pFR293), GST-Akl1(767-1108; S1072A) (pFR294), or GST-Akl1(767-1108; S960A S1072A) (pFR297), also purified from E. coli. The resulting products were resolved by SDS-PAGE and analyzed by autoradiography and staining with Coomassie dye. (C) A wild-type (WT) strain (BY4741) and an isogenic fpk1Δ fpk2Δ mutant (YFR205) expressing from the GAL1 promoter either GFP-Akl1 (pDD0938) or GFP-Akl1(S960A S1072A) (pFR303) were grown to mid-exponential phase and lysed. The resulting extracts were resolved on a Phos-tag gel and analyzed by immunoblotting with anti-GFP antibodies. (D) Otherwise wild-type cells expressing full-length Akl1-mCherry from its endogenous promoter at its normal chromosomal locus (YFR437) were grown, and extracts were prepared, treated with calf intestinal phosphatase, and then resolved and analyzed as for panel C. (E) Same as in panel C except that the strains were expressing GFP-Akl1 in which residues 30 to 751 were deleted (pFR304) or the same construct with the S960A (pFR329), S1072A (pFR328), or S960A S1072A mutations (pFR334). (F) A wild-type strain (BY4741) expressing GFP-Akl1(Δ30-751) (pFR304) was grown and extracts were prepared, treated with calf intestinal phosphatase, and then resolved and analyzed as for panel E. (G) Strains BY4741 (WT) and avo3 ΔCT TOR1-1 were transformed with GFP-Akl1(Δ30-751) (pFR304), grown to mid-exponential phase, and left untreated (−) or treated (+) with rapamycin (0.2 μM) for 10 min, which in this strain specifically inhibits TORC2, before being lysed and analyzed as for panel C. (H) avo3 ΔCT TOR1-1 cells transformed with GFP-Akl1(Δ30-751) (pFR304), GFP-Akl1(Δ30-751; S960A) (pFR329), GFP-Akl1(Δ30-751; S1072A) (pFR328), or GFP-Akl1(Δ30-751; S960A S1072A) (pFR334) were grown and analyzed as for panel G.
![FIG 2 Fpk1 phosphorylates Akl1 at S960 and S1072. (A) Schematic representation of the Akl1, Ark1, and Prk1 protein kinases. The catalytic domain (red rectangle) is near the amino terminus. Akl1 has the longest C-terminal segment, and only Akl1 contains consensus Fpk1 phospho-acceptor site motifs, both near its C terminus. (B) GST-Fpk1 (pAX15, WT) or catalytically inactive (kinase-dead [KD]) mutant GST-Fpk1(D621A) (pJY10) were purified from E. coli, incubated with [γ-32P]ATP and either GST-Akl1(767-1108) (pFR290), GST-Akl1(767-1108; S960A) (pFR293), GST-Akl1(767-1108; S1072A) (pFR294), or GST-Akl1(767-1108; S960A S1072A) (pFR297), also purified from E. coli. The resulting products were resolved by SDS-PAGE and analyzed by autoradiography and staining with Coomassie dye. (C) A wild-type (WT) strain (BY4741) and an isogenic fpk1Δ fpk2Δ mutant (YFR205) expressing from the GAL1 promoter either GFP-Akl1 (pDD0938) or GFP-Akl1(S960A S1072A) (pFR303) were grown to mid-exponential phase and lysed. The resulting extracts were resolved on a Phos-tag gel and analyzed by immunoblotting with anti-GFP antibodies. (D) Otherwise wild-type cells expressing full-length Akl1-mCherry from its endogenous promoter at its normal chromosomal locus (YFR437) were grown, and extracts were prepared, treated with calf intestinal phosphatase, and then resolved and analyzed as for panel C. (E) Same as in panel C except that the strains were expressing GFP-Akl1 in which residues 30 to 751 were deleted (pFR304) or the same construct with the S960A (pFR329), S1072A (pFR328), or S960A S1072A mutations (pFR334). (F) A wild-type strain (BY4741) expressing GFP-Akl1(Δ30-751) (pFR304) was grown and extracts were prepared, treated with calf intestinal phosphatase, and then resolved and analyzed as for panel E. (G) Strains BY4741 (WT) and avo3 ΔCT TOR1-1 were transformed with GFP-Akl1(Δ30-751) (pFR304), grown to mid-exponential phase, and left untreated (−) or treated (+) with rapamycin (0.2 μM) for 10 min, which in this strain specifically inhibits TORC2, before being lysed and analyzed as for panel C. (H) avo3 ΔCT TOR1-1 cells transformed with GFP-Akl1(Δ30-751) (pFR304), GFP-Akl1(Δ30-751; S960A) (pFR329), GFP-Akl1(Δ30-751; S1072A) (pFR328), or GFP-Akl1(Δ30-751; S960A S1072A) (pFR334) were grown and analyzed as for panel G.](/cms/asset/8229b957-1666-4523-bff3-1b29916fdd99/tmcb_a_12276196_f0002_oc.jpg)
FIG 3 Sphingolipids stimulate Fpk1 function by a mechanism distinct from alleviation of TORC2-Ypk1-mediated inhibition. (A) A wild-type strain (BY4741) and an isogenic fpk1Δ fpk2Δ mutant (YFR205) expressing from the GAL1 promoter GFP-Akl1(pDD0938) were grown to mid-exponential phase, and expression was induced with galactose. After 1 h of induction, cells were treated with a vector (−), 1.25 μM myriocin (Myr), or 10 μM phytosphingosine (PHS) for 2 additional hours. The cells were then lysed and analyzed as for . (B) Same as in panel A, except with WT cells, slm1Δ slm2Δ cells expressing Ypk1D242A (YFR381), and ypk1Δ cells. (C) Anti-Ypk1 phospho-T662 antibodies recognize the TORC2-phosphorylated forms of endogenous Ypk1 and Ypk2 and plasmid-expressed Ypk1-3×HA. Wild-type (BY4741) or otherwise isogenic ypk1Δ or ypk2Δ cells expressing Ypk1-3×HA (pPL215) were grown to mid-exponential phase and then treated with either a vehicle (methanol) or 1.25 μM myriocin for 2 h to induce TORC2 activation prior to harvesting. Whole-cell extracts were prepared, resolved by Phos-tag SDS-PAGE, and analyzed by immunoblotting with anti-Ypk1 phospho-T662 antibodies and anti-HA.11 epitope antibody. (D) Same as in panel C except that wild-type (BY4741) or slm1Δ slm2Δ (yKL28) cells expressing Ypk1(D242A)-3×HA (pKL27) were used.
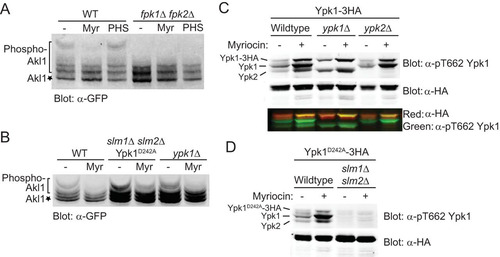
FIG 4 Phosphorylation by Fpk1 downregulates Akl1 activity. (A) Sequence of Sla1. Shown are the consensus Akl1/Prk1 phospho-acceptor site (yellow box), matching either the Prk1 motif (L/I/V/MXXQ/N/T/SXTG) determined in Pan1 (Citation51) or the Akl1 motif (L/I/V/MXXQ/H/M/T/N/AXTG) determined using synthetic peptide arrays (Citation35), and the consensus Ypk1 phospho-acceptor sites (turquoise box), RXRXXS[Φ] (where “[Φ]” indicates a preference for a hydrophobic amino acid) (Citation8). (B) Cultures of an akl1Δ mutant (YFR479) expressing either Akl1-3×FLAG (pFR316) or a catalytically inactive (kinase-dead) mutant, Akl1(D181Y)-3×FLAG (pFR318), were lysed and the corresponding 3×FLAG-tagged proteins recovered by immunoprecipitation with mouse anti-FLAG antibodies. The resulting immunoprecipitates were incubated with [γ-32P]ATP and GST-Sla1(854-918) that had been purified from E. coli harboring plasmid pDD0214. The resulting products were resolved by SDS-PAGE and analyzed as described in Materials and Methods. (C) Same as in panel B except that an akl1Δ mutant (YFR479) expressing either Akl1-3×FLAG (pFR316) or Akl1AA-3×FLAG (pFR319) was used. Numbers beneath each time point represent the autoradiogram/Coomassie signals, normalized to the ratio observed for WT Akl1 at 15 min. (D) The immunoprecipitates obtained for panel C were resolved by SDS-PAGE and analyzed by immunoblotting with anti-FLAG antibodies. (E) A wild-type (WT) strain (BY4741), an isogenic fpk1Δ fpk2Δ mutant (YFR205), and strains expressing Akl1 (YFR507) or Akl1AA (YFR508) and expressing from the GAL1 promoter 3×FLAG-Sla1(851-1244) (pFR360) were grown to mid-exponential phase, and expression was induced with galactose for 3 h. The cells were harvested and lysed, trichloroacetic acid extracts were prepared, and the precipitated proteins were resolubilized, treated with calf intestinal phosphatase (CIP), resolved by SDS-PAGE, and analyzed by immunoblotting. (F) Strains expressing Ent2-GFP (YFR491-A) and Ent2-GFP fpk1Δ fpk2Δ (YFR492-A) were grown to mid-exponential phase, and cells were harvested and lysed. The resulting extracts were resolved in duplicate on a Phos-tag gel (the rightmost pair of lanes were loaded with 25% more sample than the leftmost pair of lanes) and analyzed by immunoblotting with anti-GFP antibodies.
![FIG 4 Phosphorylation by Fpk1 downregulates Akl1 activity. (A) Sequence of Sla1. Shown are the consensus Akl1/Prk1 phospho-acceptor site (yellow box), matching either the Prk1 motif (L/I/V/MXXQ/N/T/SXTG) determined in Pan1 (Citation51) or the Akl1 motif (L/I/V/MXXQ/H/M/T/N/AXTG) determined using synthetic peptide arrays (Citation35), and the consensus Ypk1 phospho-acceptor sites (turquoise box), RXRXXS[Φ] (where “[Φ]” indicates a preference for a hydrophobic amino acid) (Citation8). (B) Cultures of an akl1Δ mutant (YFR479) expressing either Akl1-3×FLAG (pFR316) or a catalytically inactive (kinase-dead) mutant, Akl1(D181Y)-3×FLAG (pFR318), were lysed and the corresponding 3×FLAG-tagged proteins recovered by immunoprecipitation with mouse anti-FLAG antibodies. The resulting immunoprecipitates were incubated with [γ-32P]ATP and GST-Sla1(854-918) that had been purified from E. coli harboring plasmid pDD0214. The resulting products were resolved by SDS-PAGE and analyzed as described in Materials and Methods. (C) Same as in panel B except that an akl1Δ mutant (YFR479) expressing either Akl1-3×FLAG (pFR316) or Akl1AA-3×FLAG (pFR319) was used. Numbers beneath each time point represent the autoradiogram/Coomassie signals, normalized to the ratio observed for WT Akl1 at 15 min. (D) The immunoprecipitates obtained for panel C were resolved by SDS-PAGE and analyzed by immunoblotting with anti-FLAG antibodies. (E) A wild-type (WT) strain (BY4741), an isogenic fpk1Δ fpk2Δ mutant (YFR205), and strains expressing Akl1 (YFR507) or Akl1AA (YFR508) and expressing from the GAL1 promoter 3×FLAG-Sla1(851-1244) (pFR360) were grown to mid-exponential phase, and expression was induced with galactose for 3 h. The cells were harvested and lysed, trichloroacetic acid extracts were prepared, and the precipitated proteins were resolubilized, treated with calf intestinal phosphatase (CIP), resolved by SDS-PAGE, and analyzed by immunoblotting. (F) Strains expressing Ent2-GFP (YFR491-A) and Ent2-GFP fpk1Δ fpk2Δ (YFR492-A) were grown to mid-exponential phase, and cells were harvested and lysed. The resulting extracts were resolved in duplicate on a Phos-tag gel (the rightmost pair of lanes were loaded with 25% more sample than the leftmost pair of lanes) and analyzed by immunoblotting with anti-GFP antibodies.](/cms/asset/2b70b598-8054-4e86-be51-9e5bbc5cb063/tmcb_a_12276196_f0004_oc.jpg)
FIG 5 Fpk1 phosphorylation does not affect the stability or localization of Akl1. (A) Extracts from cells expressing Akl1-mCherry (YFR437), Fpk111A Akl1-mCherry (YFR468) (Fpk111A is hyperactive because no longer submitted to negative regulation by Gin4 [Citation31]), and fpk1Δ fpk2Δ Akl1-mCherry (YFR469) were resolved by standard SDS-PAGE and analyzed by immunoblotting with anti-RFP antibodies. (B) Extracts from cells expressing Akl1-3×FLAG (YFR474-A), Fpk111A-3×FLAG (YFR475-A), and Akl1EE-3×FLAG (YFR476-A) were resolved by SDS-PAGE and analyzed by immunoblotting with anti-FLAG antibodies. (C) The same strains as in panel A were examined by fluorescence microscopy.
![FIG 5 Fpk1 phosphorylation does not affect the stability or localization of Akl1. (A) Extracts from cells expressing Akl1-mCherry (YFR437), Fpk111A Akl1-mCherry (YFR468) (Fpk111A is hyperactive because no longer submitted to negative regulation by Gin4 [Citation31]), and fpk1Δ fpk2Δ Akl1-mCherry (YFR469) were resolved by standard SDS-PAGE and analyzed by immunoblotting with anti-RFP antibodies. (B) Extracts from cells expressing Akl1-3×FLAG (YFR474-A), Fpk111A-3×FLAG (YFR475-A), and Akl1EE-3×FLAG (YFR476-A) were resolved by SDS-PAGE and analyzed by immunoblotting with anti-FLAG antibodies. (C) The same strains as in panel A were examined by fluorescence microscopy.](/cms/asset/f0e1019c-2f5a-4ca3-9a9a-802ddbfc6ab5/tmcb_a_12276196_f0005_oc.jpg)
FIG 6 Lack of negative regulation of Akl1 by Fpk1 impedes endocytosis. (A) Cultures of an akl1Δ strain (YFR479) containing either an empty vector (V; YCpUG) or expressing from the GAL1 promoter in the same vector either GFP-Akl1AA (pFR303) or a catalytically inactive (kinase-dead) derivative, GFP-Akl1AA(D181A) (pKL31), were induced on galactose medium for 2.5 h, incubated with 4 mg/ml of Lucifer yellow CH (LY) for 30 min at 24°C, and then viewed directly by fluorescence microscopy. (B) Cells (YFR515) expressing both Akl1-mCherry and Sla1-GFP from their endogenous promoters at their normal chromosomal loci were grown on YPD and viewed by fluorescence microscopy. (C) Strains expressing Akl1 Sla1-GFP Abp1-RFP (WT, YFR507) and Akl1AA Sla1-GFP Abp1-RFP (YFR508) were examined by fluorescence video microscopy (see Movies S1 and S2 in the supplemental material), and kymographs were plotted as described in Materials and Methods. (D) The mean Sla1-GFP lifetime at the cell cortex was measured from multiple kymographs as for panel C. (E) The total number of Sla1-GFP patches per cell subsequently joined by Abp1-RFP and moved toward the cell center, a hallmark of actin-driven internalization (Citation40), was determined in cells as in panel C. n, total number cells examined; ns, not significant.
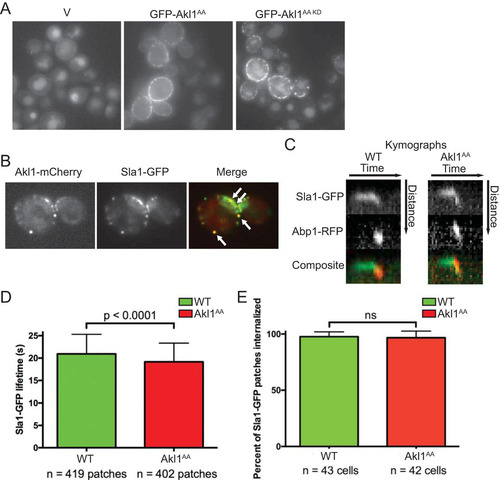
FIG 7 Fpk1 effects on both Akl1 and flippase function influence drug sensitivity. (A) Serial 10-fold dilutions of BY4741 (WT), akl1Δ (JTY6532), Fpk111A-expressing (YJW2), fpk1Δ fpk2Δ (YFR205), and dnf1Δ dnf2Δ dnf3Δ (PFY3272C) cells were spotted on plates lacking (−) or containing (+) doxorubicin (86 μM). The plates were scanned after incubation for 2 days at 30°C. (B) Same as in panel A, except with cells expressing Akl1-3×FLAG (YFR474-A), Akl1AA-3×FLAG (YFR475-A), and Akl1EE-3×FLAG (YFR476-A) and with a different stock of doxorubicin (which is light sensitive). (C) Serial 10-fold dilutions of akl1Δ (YFR479) cells carrying pRS315 (empty vector) or expressing from the same vector Akl1-3×FLAG (pFR316) or Akl1AA-3×FLAG (pFR319) were spotted on plates lacking or containing hygromycin B (70 μM). The plates were scanned after incubation for 2 days at 30°C. (D) Serial 10-fold dilutions of akl1Δ (YFR479) cells carrying YCpUG (empty vector) or expressing from the GAL1 promoter GFP-Akl1 (pDD0938) or GFP-Akl1AA (pFR303) were spotted on plates containing dextrose (Dex) or galactose (Gal), lacking or containing 0.8 μM myriocin (+Myr) as indicated. The plates were scanned after incubation for 3 days at 30°C.
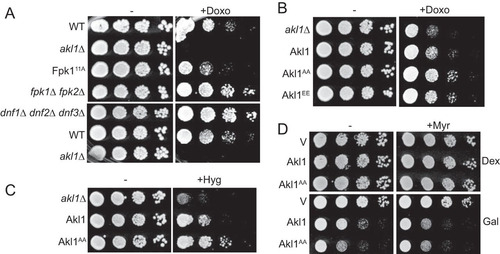
FIG 8 Fpk1 protein kinase is a signaling node that controls membrane permeability and the efficiency of endocytosis. In cells stressed by a diminution in the supply of sphingolipids, Fpk1 is less active for two reasons: (i) lack of direct stimulation by MIPC (Citation23) and (ii) inhibitory phosphorylation by Ypk1, which is activated in a TORC2-dependent manner when sphingolipids are limiting (Citation6, Citation7). The cell cycle-regulated protein kinase Gin4 also inhibits Fpk1; thus, Fpk1 is also downregulated when Gin4 is active (Citation31). Fpk1, in turn, phosphorylates and stimulates the flippases Dnf1 and Dnf2 and, as shown in this study, phosphorylates and negatively regulates Akl1. One function of Akl1 is to impede endocytosis by phosphorylation of multiple endocytic factors (including Sla1, Ent2, and Pan1), which disables their function. Thus, when Fpk1 activity is high, Akl1 function is downregulated and endocytosis can proceed; when Fpk1 activity is low, Akl1 function is released from inhibition, causing phosphorylation of it targets and thereby down-modulating the efficiency of endocytosis. Two concomitant changes in PM composition ensue when Fpk1 activity is decreased: aminoglycerophospholipid content in the outer leaflet remains higher due to lack of Fpk1-mediated stimulation of the flippases, and bulk membrane and lipid internalization via clathrin-mediated endocytosis is impeded. Both effects contribute to conferring elevated resistance to certain toxic xenobiotic compounds, such as doxorubicin and hygromycin B.
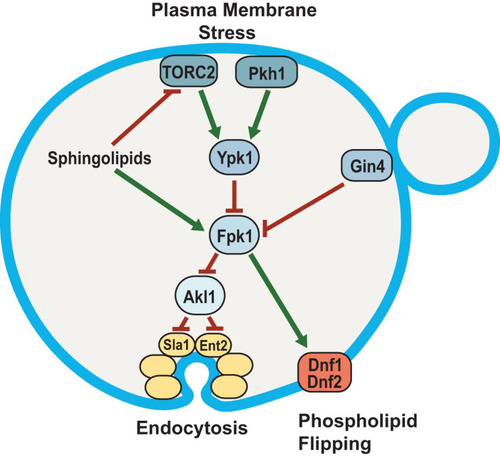
TABLE 2 S. cerevisiae strains used in this study
TABLE 3 Plasmids used in this study