Figures & data
Figure 1. Model for cutaneous immune responses at different levels of barrier disruption. (A) Application of antigen to intact skin or mildly disrupted stratum corneum leads to uptake of antigen by epidermal LCs under steady-state conditions. LC can acquire antigen from the superficial epidermis by extruding dendrites through intercellular spaces. LC continuously traffic from the skin to draining LNs through afferent lymphatics, where they interact with naïve T cells. In the absence of immunological danger signals, LC preferentially prime induction of regulatory T cells. It is unclear if, and to what extent, LC directly prime naïve T cells in the skin. (B) Epidermal antigen application on barrier-disrupted skin. Superficial epidermal trauma leads to activation of skin keratinocytes which release cytokines, such as TSLP, IL-25 and IL-33. DETCs release stress signals, such as Rae-1 (in mice) or MICA (in humans), which induce keratinocytes to secret TH2 cytokines IL-4, -13 and -25. Antigen and cytokines can also reach the dermis through diffusion. This cytokine milieu instructs LC and dDC to prime TH2 responses in naïve T cells. Whether dDC directly activate naïve T cells in the dermis is not clear. (C) Deep dermal trauma allows direct access of antigen to the dermal layer and hence uptake by dDC. The DAMPs released from destroyed cells activates keratinocytes to release IL-6, IL-1β and TGF-β, cytokines instructing dDC to prime TH17 responses. Administration of antigen to the dermis also facilitates passive drainage of antigen via the afferent lymphatics and release of antigen into the circulation.
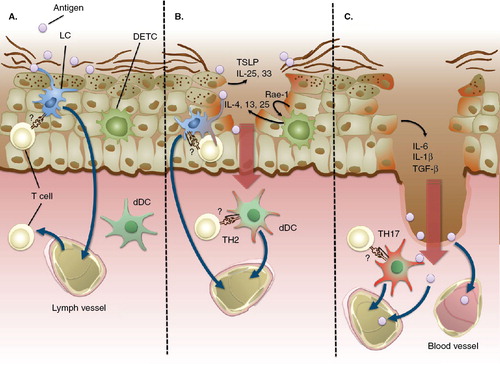
Figure 2. Histological analysis of laser-generated micropores in mouse skin. (A) Top view of skin after laserporation using 2 pulses (F = 1.9 J/cm2/pulse), 400 pores/cm2. Panels (B)–(D) show representative H&E-stained paraffin skin sections displaying a single pore after laserporation with 1 (B), 4 (C) or 8 pulses (D) delivered at 1.9 J/cm2/pulse. Panel (E) shows a SEM picture of a single pore generated by delivery of 8 pulses at 0.76 J/cm2/pulse.
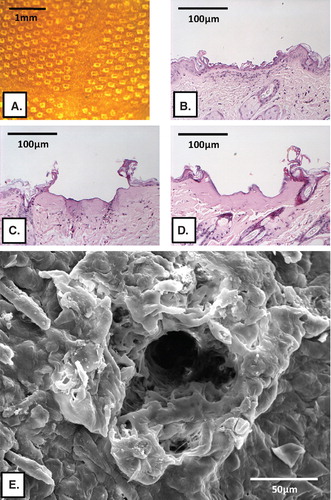
Figure 3. In vivo uptake of FITC-BSA via laser-generated micropores in mouse skin. A 5 × 5 mm piece of gauze was soaked with 80 μL of FITC-labeled BSA (10 mg/ml), applied to laser-generated micropores and covered with Tegaderm™. At different time points, skin areas were excised, embedded in Tissue-Tek® O.C.T.™ compound, and frozen sections (10 μM) were prepared. Fluorescence microscopy revealed that FITC-BSA specifically attaches to micropores, recognizable as indentations in the skin (indicated by arrows), from where it slowly diffuses into deeper skin layers. After 4 days, a residual depot is still discernable.
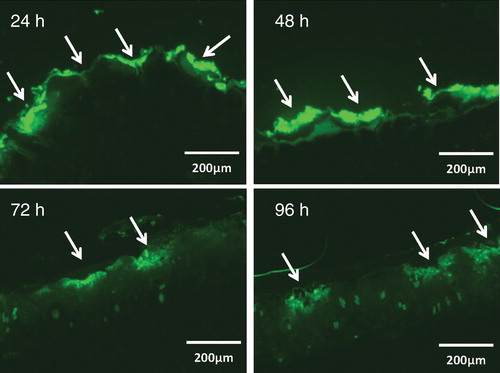
Figure 4. Prophylactic vaccination against allergy. BALB/c mice (n = 5) were vaccinated transcutaneously via laser-generated micropores or by s.c. injection with or without CpG-ODN, or with CpG-ODN alone (CpG; applied transcutaneously) and subsequently sensitized by intraperitoneal injection of alum-adsorbed allergen, followed by intranasal allergen challenge. Non-prevaccinated, but sensitized animals (‘control') and naïve animals served as controls. Allergen-specific IgE (A), the number of IL-4 secreting cells of restimulated splenocytes (B) and numbers of eosinophils in BALF (C) are shown.
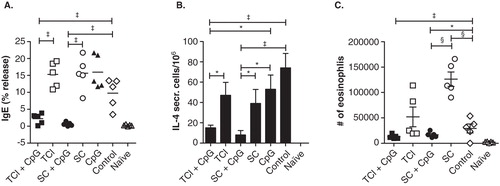
Figure 5. SIT of allergy. (A) AHR after immunotherapy of Phl p 5-sensitized mice. AHR was assessed before and after TCIT or SCIT via whole-body plethysmography or by invasive measurement of lung resistance and dynamic compliance. Data are shown as individual data points or means ± SEM (n = 12). BL: baseline. (B) Cellular composition in BALF and (C) TH2 cytokine secretion of restimulated splenocytes after immunotherapy of Phl p 5-sensitized mice. Data are shown as individual data points and/or means ± SEM (n = 12) of mice treated by TCIT or SCIT or untreated control mice.
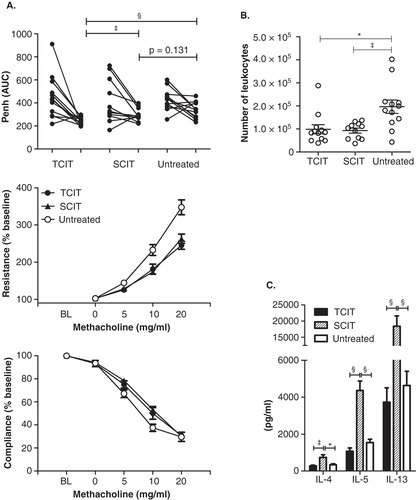