Figures & data
Figure 1 General overview of the epigenetic mechanisms involved in gene expression associated with cancer. Epigenetic modifications in DNA, histones, and the biosynthesis of Non-coding RNAs (ncRNAs) are closely related to the genesis of several types of tumors. These modifications also contribute to regulating other key events in the establishment of the disease, such as cell proliferation, cell cycle changes, apoptosis, invasion, metastasis, DNA damage and senescence. DNA and histone methylation, histone acetylation and ncRNA biosynthesis can activate oncogenes and repress tumor suppressor genes, often related to tumorigenesis. Thus, enzymes that catalyze epigenetic modifications, such as DNA methyltransferases (DNMTs), ten-eleven translocation enzymes (TETs), histone acetylases (HATs), histone deacetylases (HDACs), histone methyltransferases (HMTs) and histone demethylases (HDMs), are the main agents that promote epigenetic modifications in DNA and histones, capable of inducing reversible chromatin remodeling, and activating or repressing the transcription of cancer-related genes.
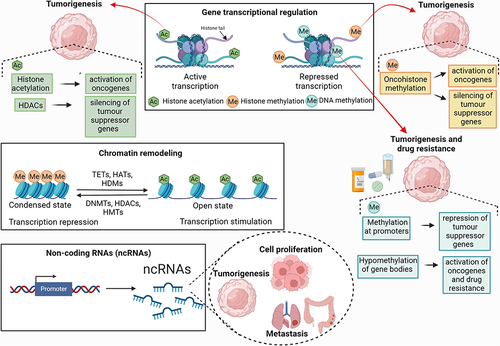
Table 1 Some of the Main Epigenetic Biomarkers Involved in the Immune Control of Tumors
Table 2 Some Immunotherapy Treatments, Their Associated Epigenetic Hallmarks Found in Cancer Patients and Clinical Outcomes
Figure 2 Main immunotherapy targets and therapeutic effects for the tumor microenvironment (TME) at the cellular level. The expression of genes related to the immune system can be modulated by DNA and histone methylation. 1) Hypomethylation of immunological synapse genes (CD40, CD80 and CD86) of 20 human solid tumors, such as melanoma, by DNMT1 demonstrated correlation with modulation of TME tolerogenicity by infiltration of CD4+ and CD8+ T cells. In different ovarian cancers, overexpression, and silencing of the Ccl5 gene, respectively by demethylation and hypermethylation mediated by DNMT1, can modulate tumor infiltration by CD8+ T cells. In human ovarian cancer, overexpression of Ccl5 leads to increased tumor immunoinfiltration. In ID8 ovarian tumors in mice, Ccl5 silencing by hypermethylation is related to deficiency in both tumor-infiltrating lymphocyte recruitment (TILs) and Chemokine (C-X-C motif) ligand 9 (CXCL9) secretion by tumor-associated macrophages (TAMs). 2) Antigen presentation, necessary for the antitumor cytotoxic response, can be inhibited by EZH2-mediated histone methylation. In human melanoma, EZH2 inhibition and consequent upregulation of the HLA-I gene increased the recruitment and infiltration of CD8+PD-1+ T cells and CD20+ B cells into the TME. 3) Both DNA and histone methylations, respectively by DNMT and EZH2, result in partial or total downregulation of chemoattractant cytokine genes (Th1 Cks) Cxcl9 and Cxcl10, decreasing recruitment of CD8+ T cells to the TME in mouse ovarian tumors.
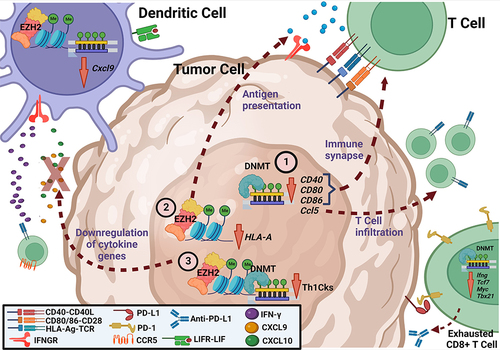
Figure 3 Examples of approaches available to increase the effectiveness of immuno-oncology therapies. (A) use of immune checkpoint inhibitors to target and destroy tumor cells; (B) anticancer immunotherapy based on the use of the patient’s own genetically modified immune cells; (C) combination of two complementary anticancer therapies; (D) administration of anticancer vaccines in either a prophylactic or therapeutic approach; (E) use of oncolytic viruses, cytokines and colony stimulating factors to make the TME more conducive to immune cell infiltration; (F) genomic investigation of tumors for the personalized choice of anticancer immunological strategies; (G) prediction of antitumor responses through the detection and characterization of biomarkers; (H) use of immune cells to specifically target cancer cells; (I) use of CAR-T cells, T-cell receptor (TCR) gene therapy, and bispecific antibodies to activate and reprogram the immune system against cancer cells and (J) identification of targets in the specific metabolism of tumor cells to make them more recognizable by components of the immune system.
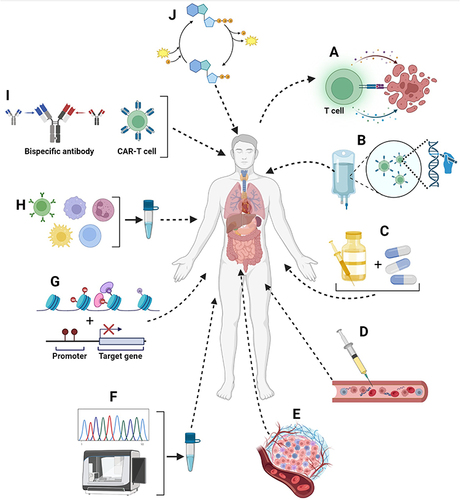