Figures & data
Figure 1 NADPH production from the oxidative PPP and one-carbon serine catabolism pathway.
Abbreviations: PPP, pentose phosphate pathway; NADPH, nicotinamide adenine dinucleotide phosphate; G6PD, glucose-6-phosphate dehydrogenase; 6PGD, 6-phosphogluconate dehydrogenase; 6-AN, 6-aminonicotinamide; NMN, nicotinamide mononucleotide; NAMPT, nicotinamide phosphoribosyltransferase; NADK, NAD+-kinase; ROS, reactive oxygen species; TIGAR, TP53-induced glycolysis and apoptosis regulator; PKM2, pyruvate kinase 2; G6P, glucose-6-phosphate; 6PG, 6-phosphogluconate; R5P, ribulose-5-phosphate; F16BP, fructose-1,6-bisphosphate; PEP, phosphoenolpyruvate; FDA, food and drug administration; NAD, nicotine adenine dinucleotide.
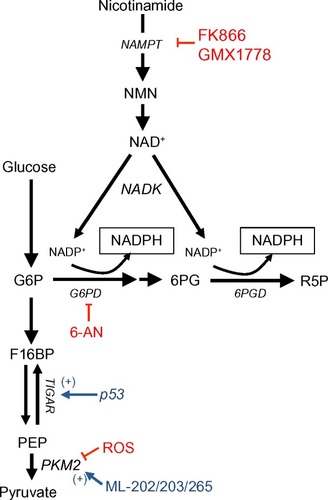
Figure 2 Agents targeting specific NADPH-biogenesis pathways.
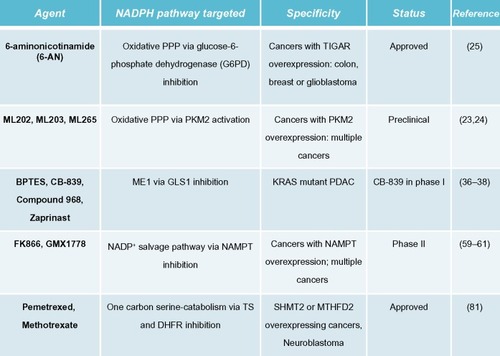
Figure 3 One-carbon serine catabolism pathway.
Abbreviations: NADPH, nicotinamide adenine dinucleotide phosphate; MTHFD2, methylenetetrahydrofolate dehydrogenase; THF, tetrahydrofolate; DHF, dihydrofolate; DHFR, dihydrofolate reductase; PEM, pemetrexed; MTX, methotrexate; dUMP, deoxyuridine monophosphate; TS, thymidylate synthase; SHMT2, serine hydroxymethyltransferase 2.
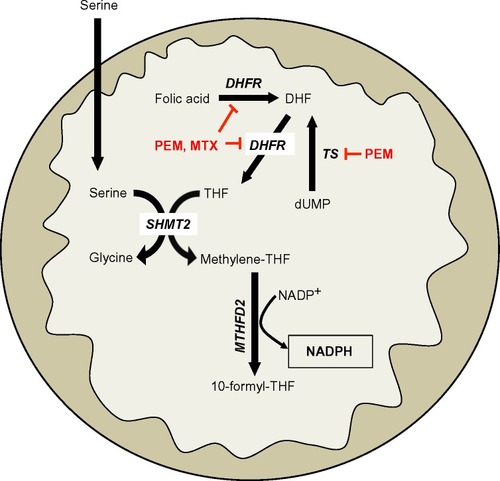
Figure 4 KRAS-reprogrammed glutamine metabolism in pancreatic cancer.
Abbreviations: GOT2, mitochondrial aspartate transaminase; GLUD1, glutamate dehydrogenase 1; Asp, aspartate; NADPH, nicotinamide adenine dinucleotide phosphate; ME1, malic enzyme 1; ME2, malic enzyme 2; BPTES, bis-2-(5-phenylacetamido-1,2,4-thiadiazol-2-yl)ethyl sulfide 3; GLS1, glutaminase 1; MDH1, malate dehydrogenase 1; GOT1, cytosolic aspartate transaminase; OAA, oxaloacetate; αKG, α-ketoglutarate; TCA, tricarboxylic acid cycle.
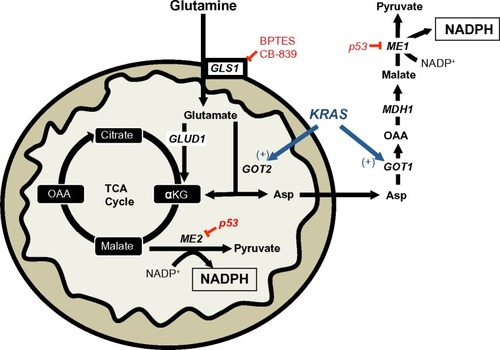
Figure 5 NADPH biogenesis via IDH1.
Abbreviations: IDH1, isocitrate dehydrogenase 1; NADPH, nicotinamide adenine dinucleotide phosphate; IDH2, isocitrate dehydrogenase 2; αKG, α-ketoglutarate.
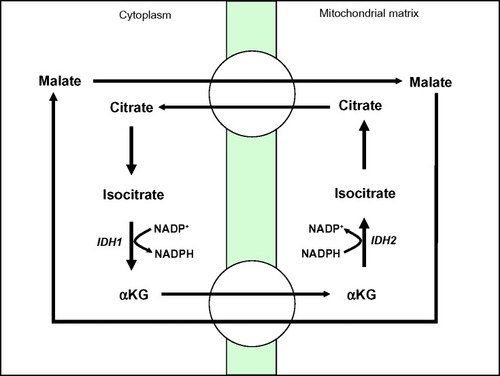
Figure 6 Work flow to identify NSCLC-specific NADPH-biogenesis genes in a cancer-specific NADPH-biogenesis screen.
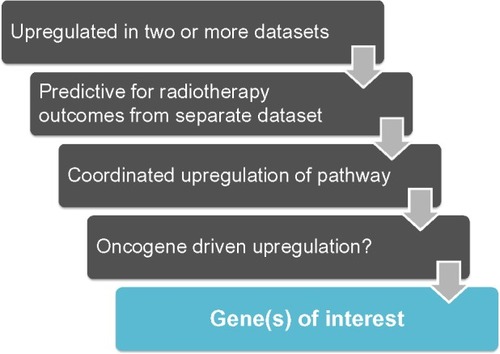
Figure 7 A cancer-specific NADPH-biogenesis screen.
Abbreviations: NADPH, nicotinamide adenine dinucleotide phosphate; NSCLC, non-small cell lung cancer; G6PD, glucose-6-phosphate dehydrogenase; PKM2, pyruvate kinase 2; TIGAR, TP53-induced glycolysis and apoptosis regulator; SHMT2, serine hydroxymethyltransferase 2; MTHFD2, methylenetetrahydrofolate dehydrogenase; ME1, malic enzyme 1; ME2, malic enzyme 2; IDH1, isocitrate dehydrogenase 1; IDH2, isocitrate dehydrogenase 2; NAMPT, nicotinamide phosphoribosyltransferase.
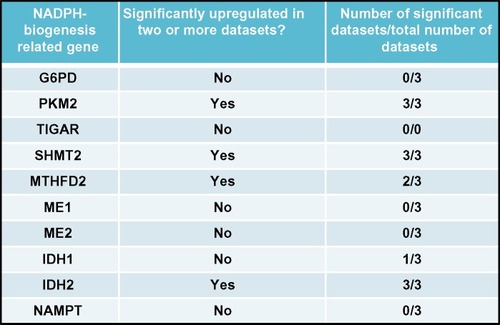
Figure 8 Cancer-specific NADPH-biogenesis screen continued.
Abbreviations: NADPH, nicotinamide adenine dinucleotide phosphate; NSCLC, non-small cell lung cancer; MTHFD2, methylenetetrahydrofolate dehydrogenase; PKM2, pyruvate kinase 2; SHMT2, serine hydroxymethyltransferase 2; IDH2, isocitrate dehydrogenase 2; G6PD, glucose-6-phosphate dehydrogenase; TS, thymidylate synthase.
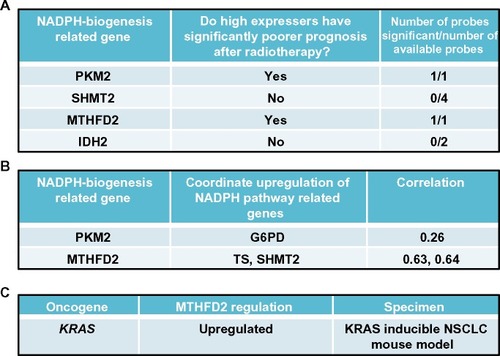
Figure 9 Screen results for MTHFD2 in NSCLC patients.
Abbreviations: MTHFD2, methylenetetrahydrofolate dehydrogenase; NSCLC, non-small cell lung cancer; NADPH, nicotinamide adenine dinucleotide phosphate; SHMT2, serine hydroxymethyltransferase 2; TS, thymidylate synthase; HR, hazard ratio.
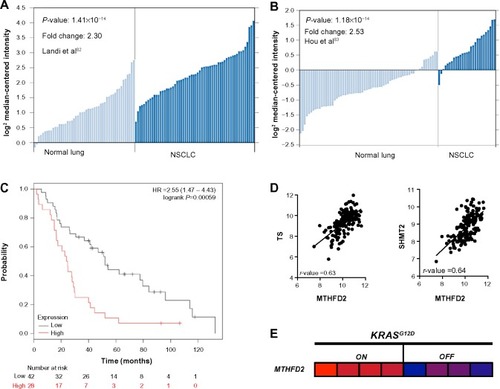