Figures & data
Figure 1 Interaction of bacteria and mammalian cells with nanoparticles.
Notes: (A) Schematics of the effects of nanoparticles on bacterial cells. (B and C) Representative electron microscopy images showing the interaction of E. coli with the samples of cerium oxide nanoparticles at different magnifications. (D) Programmable nanomaterial synthesis using GNPs under different deposition parameters. (E) Effects of CeO2 nanoparticles on cardiac progenitor cell morphology and growth. CeO2-untreated (a) or CeO2-treated cells 10 μg/mL (b), 25 μg/mL (c) and 50 μg/mL (d). (A–C) Republished with permission of American Society for Microbiology, from Effects of engineered cerium oxide nanoparticles on bacterial growth and viability. Pelletier DA, Suresh AK, Holton GA, et al. Appl Environ Microbiol. 76(24):7981–7989. Copyright 2010.Citation167 (D) Reprinted by permission from Springer Nature: Nat Nanotechnol. Programming the detection limits of biosensors through controlled nanostructuring. Soleymani L, Fang Z, Sargent EH, Kelley SO. Copyright 2009.Citation168 (E) Reprinted with permission from Pagliari F, Mandoli C, Forte G, et al. Cerium oxide nanoparticles protect cardiac progenitor cells from oxidative stress. ACS Nano. 2012;6(5):3767–3775. Copyright 2012 American Chemical Society.Citation169
Abbreviations: E. coli, Escherichia coli; GNP, gold nanoparticle; ROS, reactive oxygen species.
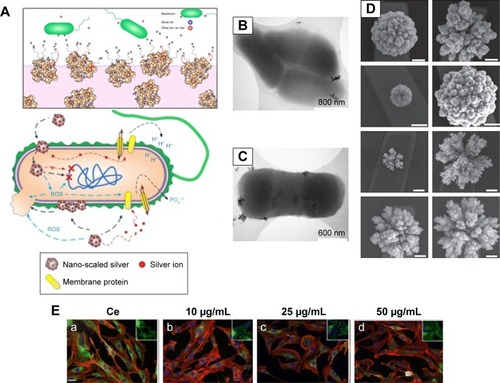
Table 1 Electrical conductivity of nanocomposites and their applications in the biomedical field
Figure 2 Antimicrobial activity of nanoparticles on bacteria.
Notes: (A) Antibacterial nanoscaffolds with silver nanoparticles. (B) (i) Simultaneous fluorescent and transmission image of E. coli culture mixed with aerogel prepared MgO/fluorescein (the fluorescent areas are green; the nonfluorescent areas are red). (ii and iii) AFM images. (iv and v) Cross sections and their section z-heights below. (C) Photothermal inactivation of heat-resistant bacteria on nanoporous gold disk arrays at different magnifications and array structures (i–vi). Reprinted with permission from Optical Society of America. Santos GM, de Santi Ferrara FI, Zhao G, Rodrigues DF, Shih W. Photothermal inactivation of heat-resistant bacteria on nanoporous gold disk arrays. Opt Mater Express. 2016;6(4):1217–1229.Citation170 (D) Transmission electron micrographs of Bacillus incubated with 5 μg⋅mL−1 mercaptopropionic acid–GNP (i and ii), 5 μg⋅mL−1 cationic 3-mercaptopropylamine–GNP (iii and iv) and 0.5 μg⋅mL−1 cationic polyelectrolyte poly(allylamine hydrochloride)–GNP (v and vi). White arrows in figure D point to binding sites of NPs with cells; yellow arrows denote lysed cells or empty cells; dashed arrows show cell wall-free cytoplasmic content. (E) Novel selenium nanoparticles killing E. coli when coated on polyvinyl chloride. Arrows indicate bacteria. (F) The use of iron oxide MNPs and a magnet to penetrate an E. coli biofilm and kill bacteria (as indicated by the red dead stain; live bacteria are stained green). (i) Iron oxide nanoparticles coated in polymersomes. Scale bar=1 μm. (ii) Bright-field image showing the placement of a black magnet under the well plate (left) and (iii) the same area under fluorescent imaging showing red (dead) bacteria in the exact same area. Scale bar=50 μm. (A–B) Reprinted with permission from Stoimenov PK, Klinger RL, Marchin GL, et al. Metal oxide nanoparticles as bactericidal agents. Langmuir. 2002;18(17):6679–6686. Copyright 2002 American Chemical Society.Citation81 (D) Reproduced from Feng Z, Gunsolus IL, Qiu TA, et al. Impacts of gold nanoparticle charge and ligand type on surface binding and toxicity to Gram-neg ative and Gram-positive bacteria. Chem Sci. 2015;6(9):5186–5196.Citation171
Abbreviations: E. coli, Escherichia coli; GNP, gold nanoparticle; MNP, magnetic nanoparticle.
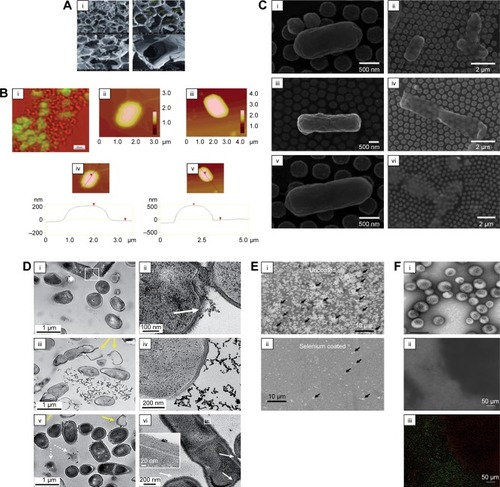
Figure 3 Utilization of magnetic particles in bioengineering applications.
Notes: (A) Upper side of the figure shows MNP-induced apoptosis, whereas the bottom side shows silver nanoparticle-induced apoptosis. (B) Transplantation procedure of MSC sheets using an electromagnet with harvesting, transportation and transplantation. (C) Principles and schematic of magnetofection. (D) Size-dependent contrast agents with their T2-weighted magnetic resonance images and color maps; the scale bar is 50 nm. (A) Reprinted from Colloids Surf B Biointerfaces. 77(2). Gopinath P, Gogoi SK, Sanpui P, Paul A, Chattopadhyay A, Ghosh SS. Signaling gene cascade in silver nanoparticle induced apoptosis. Pages 240–245., Copyright 2010, with permission from Elsevier.Citation104 (B) © 2007 Wiley Periodicals, Inc. J Biomed Mater Res Part B: Appl Biomater 2007. Reproduced from Shimizu K, Ito A, Yoshida T, Yamada Y, Ueda M, Honda H. Bone tis sue engineering with human mesenchymal stem cell sheets constructed using magnetite nanoparticles and magnetic force. Journal of Biomedical Materials Research.Citation172 (D) Reprinted from Springer Nature: Nature Medicine. Lee JH, Huh YM, Jun YW, et al. Artificially engineered magnetic nanoparticles for ultra-sensitive molecular imaging. Copyright 2007.Citation173
Abbreviations: MNP, magnetic nanoparticle; Bcl-2, B-cell lymphoma 2; MSC, mesenchymal stem cells.
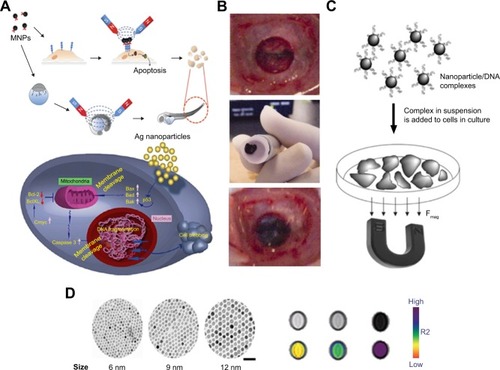
Figure 4 Magnetic cell patterning and ferrogel fabrication.
Notes: (A–C) Magnetic cell patterning using MCLs, RGD–MCLs and PEG-Mags and their resultant pattern of cells. Reprinted from Academic Press, Ito A, Kamihira M. Progress in Molecular Biology and Translational Science/Tissue Engineering Using Magnetite Nanopar ticles, pages 355–395, Copyright 2011.Citation174 (D–G) Schematic of fabrication of monophasic and biphasic ferrogels, their SEM/EDS and micro-CT images and these ferrogels in the presence of no magnetic field (field off) and a moderate vertical magnetic field gradient (field on). Reproduced from Cezar CA, Kennedy SM, Mehta M, et al. Biphasic ferrogels for triggered drug and cell delivery. Adv Healthc Mater. 2014;3(11):1869–1876. With permission from John Wiley and Sons.Citation175
Abbreviations: EDS, energy dispersive spectroscopy; MCL, magnetite cationic liposome; RGD–MCL, arginine–glycine–aspartic acid motif-containing peptide coupled to a phospholipid group on magnetite cationic liposomes; PEG-Mags, poly(ethylene glycol) in combination with a magnetic force; SEM, scanning electron microscopy; micro-CT, micro-computed tomography.
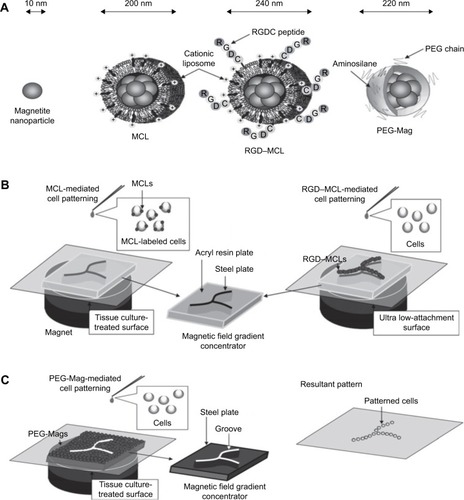
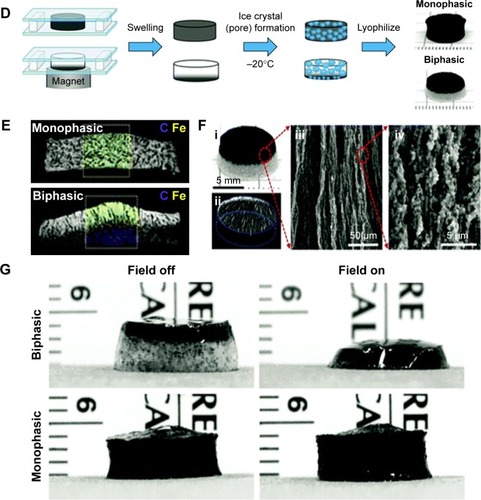
Figure 5 Magnetic culturing of cells using cell levitation and cocultures.
Notes: (A) Schematic of heterotypic cell cocultures using magnetic force. (B) Schematic of magnetic levitation in a Petri dish (left) and a 24-well plate (right). The cells are levitated to the air–liquid interface by placing a neodymium magnet above the dish or well. Cell culture under magnetic stimuli: (C) schematic drawing of the setup, (D) bright-field image and (E) fluorescence images of the two types of microcryogels (poly(ethyelene glycol) diacryate microcryogels [PM]; and magnetic gelatin microcryogels [MGM]) mixed together and co-cultured in the system. Scale bar is 200 μm. (A) Reprinted from Trends Biotechnol. 27(8). Corchero JL, Villaverde A. Biomedical applications of distally controlled magnetic nanoparticles. Pages 468–476. Copyright 2009, with permission from Elsevier.Citation176 (B) The publisher for this copyrighted material is Mary Ann Liebert, Inc. publishers. Tseng H, Gage JA, Raphael RM, et al. Assembly of a three-dimensional multitype bronchiole coculture model using magnetic levitation. Tissue Eng Part C Methods. 2013;19(9):665–675.Citation177 (C–E) Republished with permission of Royal Society of Chemistry, from Magnetically controllable 3D microtissues based on magnetic microcryogels, Liu W, Li Y, Feng S, et al, Volume 14(15), 2014; permission conveyed through Copyright Clearance Center, Inc.Citation178
![Figure 5 Magnetic culturing of cells using cell levitation and cocultures.Notes: (A) Schematic of heterotypic cell cocultures using magnetic force. (B) Schematic of magnetic levitation in a Petri dish (left) and a 24-well plate (right). The cells are levitated to the air–liquid interface by placing a neodymium magnet above the dish or well. Cell culture under magnetic stimuli: (C) schematic drawing of the setup, (D) bright-field image and (E) fluorescence images of the two types of microcryogels (poly(ethyelene glycol) diacryate microcryogels [PM]; and magnetic gelatin microcryogels [MGM]) mixed together and co-cultured in the system. Scale bar is 200 μm. (A) Reprinted from Trends Biotechnol. 27(8). Corchero JL, Villaverde A. Biomedical applications of distally controlled magnetic nanoparticles. Pages 468–476. Copyright 2009, with permission from Elsevier.Citation176 (B) The publisher for this copyrighted material is Mary Ann Liebert, Inc. publishers. Tseng H, Gage JA, Raphael RM, et al. Assembly of a three-dimensional multitype bronchiole coculture model using magnetic levitation. Tissue Eng Part C Methods. 2013;19(9):665–675.Citation177 (C–E) Republished with permission of Royal Society of Chemistry, from Magnetically controllable 3D microtissues based on magnetic microcryogels, Liu W, Li Y, Feng S, et al, Volume 14(15), 2014; permission conveyed through Copyright Clearance Center, Inc.Citation178](/cms/asset/fa97a2bd-b067-4f6d-a6ba-fe6cf7476373/dijn_a_12193941_f0005_c.jpg)
Figure 6 Biosensing principles using nanoparticles and nanomaterials.
Notes: (A) Electromechanical biodetection schematic for silver nanoparticles. (B) Quantum dot electromechanical detection of DNA strands. (C) Electrochemical detection schematic for silver nanoparticles. (D) Example of localized surface plasmon resonance modulation by altering nanoparticle compositions. (A) Reprinted by permission from Springer Nature: Anal Bioanal Chem. Aptamer-based electrochemical approach to the detection of thrombin by modification of gold nanoparticles. Li L, Zhao H, Chen Z, Mu X, Guo L. Copyright 2010.Citation179 (B) Reprinted with permission from Wang J, Liu G, Merkoçi A. Electrochemical coding technology for simultaneous detection of multiple DNA targets. J Am Chem Soc. 2003;125(11):3214–3215. Copyright 2003 American Chemical Society.Citation180 (C) Reprinted with permission from Li H, Sun Z, Zhong W, Hao N, Xu D, Chen HY. Ultrasensitive electrochemical detection for DNA arrays based on silver nanoparticle aggregates. Anal Chem. 2010;82(13):5477–5483. Copyright 2010 American Chemical Society.Citation165 (D) Reprinted with permission from Doria G, Conde J, Veigas B, et al. Noble metal nanoparticles for biosensing applications. Sensors (Basel). 2012;12(2):1657–1687.Citation181
Abbreviation: Abs, absolute.
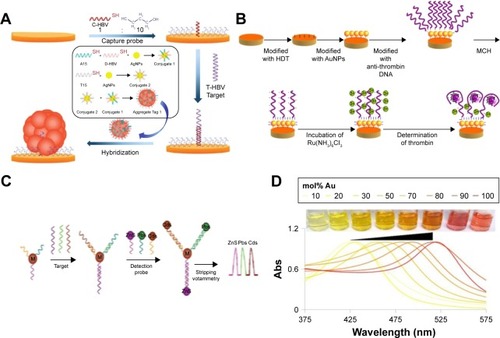