Figures & data
Figure 1 Types of angiogenesis. Types of angiogenic growth include intussusceptive (left panel) and sprouting angiogenesis (right panel). Intussusceptive angiogenesis, also known as splitting angiogenesis, represents the splitting of an existing blood vessel to form new blood vessels by a splitting process involving series of changes of interstitial tissues which invade the existing blood vessel in the presence of pericytes and fibroblasts which ends up with the core splitting by intraluminal pillars development of periendothelial cells and the formation of new smaller vessels or capillaries. Sprouting angiogenesis, as indicated from the name, involves the sprouting of endothelial cells from pre-existing blood vessel by the interruption of endothelial cells basement membrane, migration of endothelial cells along the projection’s tip via stalk cells’ formation into the surrounding connective tissue with further sprouting and new capillary network formation.
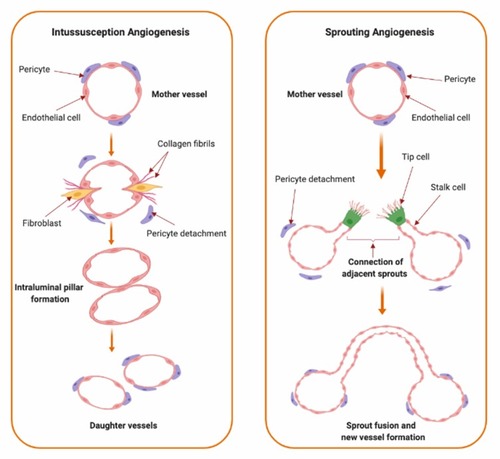
Figure 2 VEGF/VEGFR downstream signaling pathways and sites of actions of approved anti-angiogenic drugs in cancer therapy. Binding of VEGF to VEGFR leads to activation of multiple downstream cell signaling pathways including FAK/paxillins, PIP2, RAS/MAPK, and PI3K/Akt/mTOR-pathways. Monoclonal antibodies such as bevacizumab and ramucirumab bind to VEGF and VEGFR, respectively, preventing receptor activation and subsequent downstream signaling. Cabozantinib, pazopanib, regorafenib, sorafenib, sunitinib, and vandetanib are tyrosine kinase inhibitors which target the kinase domain of VEGFR. Temsirolimus and everolimus are inhibitors of mTOR which is a downstream effector for the VEGF/VEGFR axis. Collectively, these drugs will abolish angiogenic signaling in endothelial cells. These anti-angiogenic effects occur via inhibiting endothelial cells migration, vasopermeability, proliferation and survival.
Abbreviations: Akt, protein kinase B; eNOS, endothelial nitric oxide synthase; FAK, focal adhesion kinase; IP3, inositol trisphosphate; MAPK, mitogen-activated protein kinase; MEK, mitogen-activated protein kinase kinase; mTOR, mammalian target of rapamycin; PKC, protein kinase C; PIP2, phosphatidylinositol 4,5-bisphosphate; PI3K, phosphoinositide-3-kinase; RAF, rapidly accelerated fibrosarcoma; VEGF, vascular endothelial growth factor; VEGFR, vascular endothelial growth factor receptor.
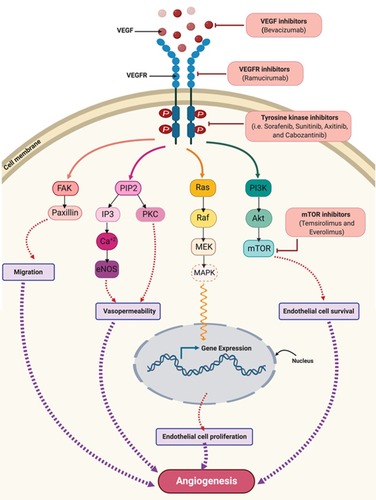
Figure 3 Schematic illustration of different shapes of a single crystalline gold nanoparticle (AuNP). Upper panel: In the simplest structure, AuNPs are commonly composed of central inner gold atoms with a closed-packed crystallographic face-centered cubic structure (domain I), surrounded by surface atoms on the outer surface of the gold atoms (domain II), which could be functionalized with an outmost layer of surface organic ligand or surfactants (domain III). The structure above is shown with a spherical shape, however, it could be also synthesized in various shapes as examples shown in 3D illustrations in the lower panel. *In the upper panel, the surface protecting ligands/surfactants were added to the posterior part of the spherical nanoparticle only for a clearer presentation of domains I and II.
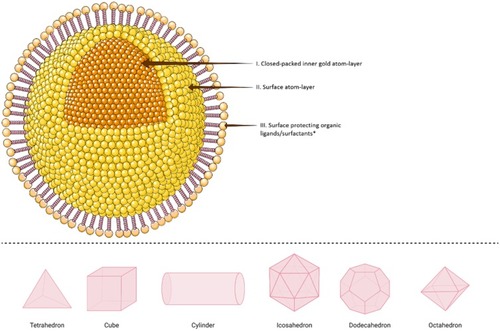
Figure 4 A schematic illustration of different AuNPs synthesis methods. (A) Turkevich method. This method uses sodium citrate as a gold-reducing agent and capping agent, (B) Brust-Schiffrin method includes the use of phase transfer agent (TOAB) and thiol-mediated capping of AuNPs, and (C) Murray method or place-exchange method, represented in organo-thiol (-SH) system, which can be used with different ligands and biomolecules.
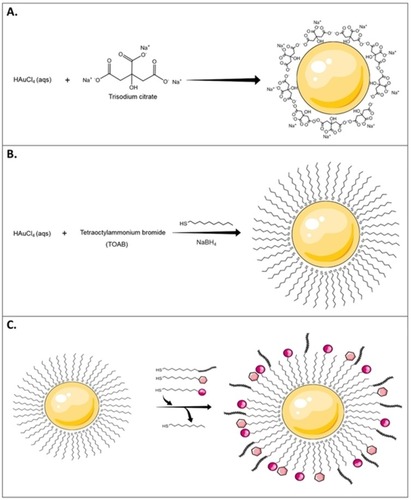
Figure 5 The seed-mediated growth synthesis method of different shapes of AuNPs. In Seed-mediated growth method, seed particles are produced by the reduction of gold salts using a small amount of a strong reducing agent (e.g. Sodium borohydride; NaBH4). Then these seeds are added to a metal salt solution in the presence of weak reducing agent (e.g. ascorbic acid) and surfactant (e.g. cetyltrimethylammonium bromide; CTAB). Changing the concentration of seed particles, reducing agents and/or the surface-protecting agents control the geometry of the resulted gold nanostructure.
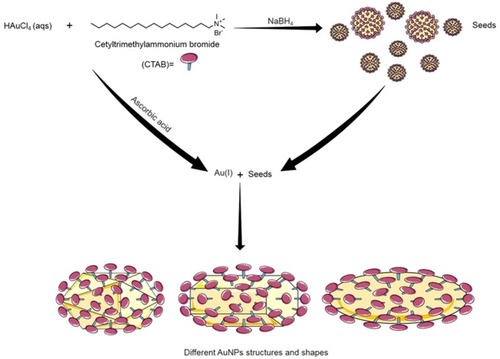
Figure 6 Internalization and cellular uptake pathways of AuNPs. Different properties of AuNPs, such as particle size, shape, surface charge, and functionalization affect internalization and cellular uptake mechanisms in different cells. These pathways include: (a) passive uptake for small AuNPs, (b) phagocytosis and (c) micropinocytosis for large size nanoparticle or aggregates of AuNPs, different pinocytosis pathways; (d) clathrin-dependent endocytosis, (e) caveolin-dependent endocytosis, and (f) clathrin/caveolin-independent endocytosis which are usually the uptake pathways for AuNPs with functionalized-, charged- or neutral-surfaces. Spherical AuNPs are used for presentation purposes only, different shapes of AuNPs are internalized by a variety of cells and affect the internalization pathways as well.
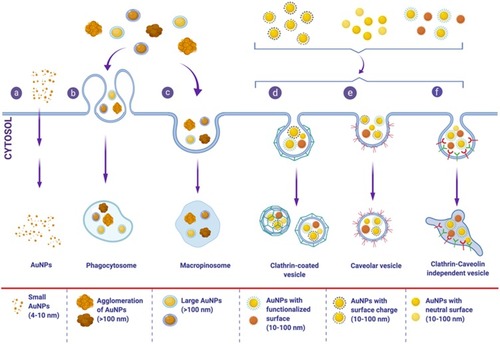
Figure 7 Molecular pathways affected by the anti-angiogenic effects of AuNPs. Anti-angiogenic effects of AuNPs are mediated by suppressing activation of VEGFR2, Tie2R, FGFR, and their downstream signaling pathways. AuNPs suppress intracellular calcium release mediated by VEGF-165. AuNPs upregulate E-cadherin and downregulate vimentin reducing epithelial-to-mesenchymal transition (EMT). AuNPs reduce ILs, MMPs, and TNF-α expression and inhibit neovascularization via induction of autophagy.
Abbreviations: Ang-1, angiopoietin 1; Ang-2, angiopoietin 2; Ca+2, calcium; FGF, fibroblast growth factor; FGFR, fibroblast growth factor receptor; ILs, interleukins; MMPs, matrix metalloproteases; Tie2R, angiopoietin receptor; TNF-α, tumor necrosis factor-α; VEGF, vascular endothelial growth factor; VEGFR, vascular endothelial growth factor receptor.
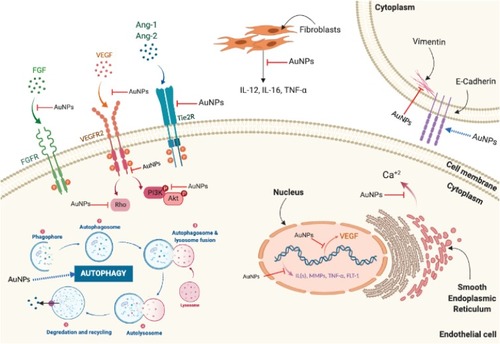