Figures & data
Figure 2 Schematic of release mechanism for glycosylated insulin from PBA-based systems upon glucose addition.
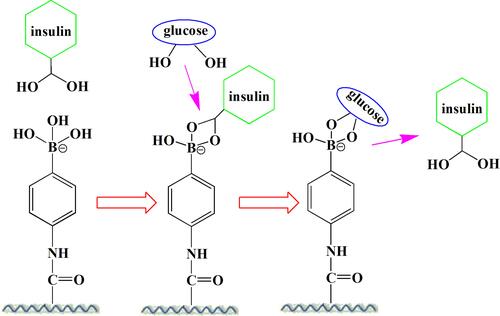
Figure 3 Schematic illustration of the formation of complex micelles of poly[(AMP)-b-(AMP-co-PBA)]-P1 and Poly[(AMP)-b-(AMP-co-GA)]-P2 or Poly[(AMP-b-poly(AMP-co-PRD)]-P3. Self-assembly of P1 was investigated at a-1 concentration of 1 mg/mL at pH 7.4 below its pKa, at which the PBA exists in the uncharged/hydrophobic form.
![Figure 3 Schematic illustration of the formation of complex micelles of poly[(AMP)-b-(AMP-co-PBA)]-P1 and Poly[(AMP)-b-(AMP-co-GA)]-P2 or Poly[(AMP-b-poly(AMP-co-PRD)]-P3. Self-assembly of P1 was investigated at a-1 concentration of 1 mg/mL at pH 7.4 below its pKa, at which the PBA exists in the uncharged/hydrophobic form.](/cms/asset/22edfe7c-dc46-4a92-b015-f3cfb0a63c28/dijn_a_12192173_f0003_c.jpg)
Figure 4 A schematic illustration of the mechanism for sugar-induced decomposition of (PBA-PAHPVA) 10 films. Sugars competitively bind to PBA-PAH in the multilayer films to replace PVA because sugars contain 1.2- and 1.3-diol moieties, resulting in destabilization or decomposition of the films.
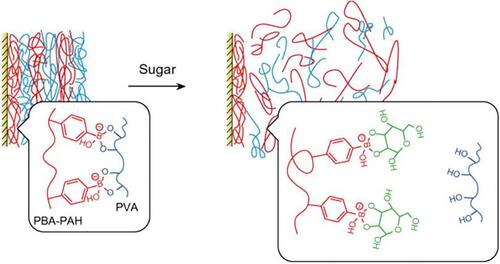
Figure 5 Cumulative release of Rd6G from MSN-PAA-AGA in PBS (pH=7.4) with different concentrations of glucose; the combination of two stimuli exhibited an obvious enhanced release capacity.
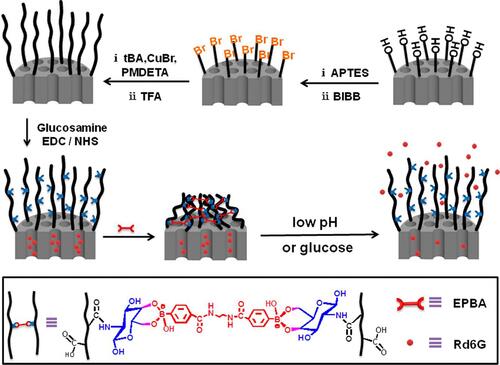
Figure 6 Schematic representation of temperature- and glucose-sensitive p(N-vinylcaprolactam -co-acrylamidophenylboronic acid) p(NVCL-co-AAPBA) nanoparticles. (A) It is a schematic diagram of nanoparticles without insulin; (B) It is a simple schematic diagram of the nanoparticle production process after adding insulin.
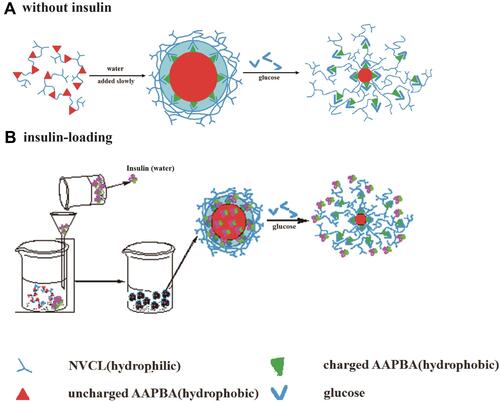
Figure 7 Schematic illustration of the glucose-responsive complex polymeric micelle (CPM) with effective glucose responsiveness and reversible swelling for repeated “on-off” release and insulin protection under physiological conditions.
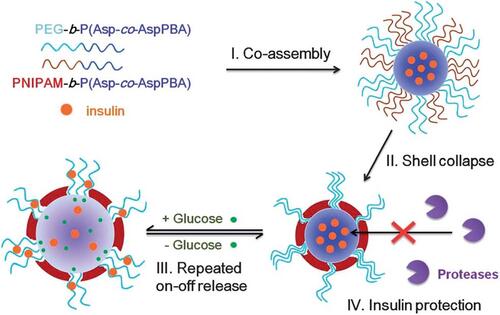
Figure 8 Expected interactions between PBA-modified insulin (PBA-Ins) and sugar chains on the cell surfaces in subcutaneous tissue and blood vessel. The cell adhesiveness of the PBA-modified drug prolongs the drug activity because the cell-attached PBA-modified drug may escape from degradation and excretion, producing slow and long-lasting activity.
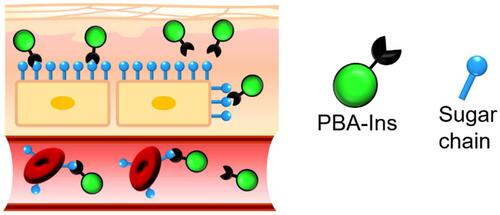
Figure 9 Schematic diagram of the reaction mechanism of p(AAPBA-b-OVZG) nanoparticles to glucose.Citation78
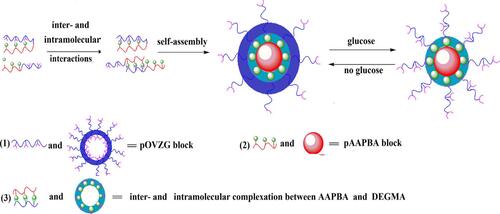
Figure 10 (A) Schematic illustration shows the pH-triggered insulin release nanomachine approach based on US-propelled mesoporous silica (MS)-Au nanomotors. (B) Glucose responsive gated insulin-containing nanocontainers. Steps involved in the insulin release mechanism: PBA-functionalized MS segment is capped with pH-sensitive nanovalves based on the GOx gating trigger molecule that leads to the autonomous insulin delivery in the presence of glucose. (C) Protonation of the PBA groups induces the opening of the pH-driven gate and uncapping of the In-loaded nanovalves.
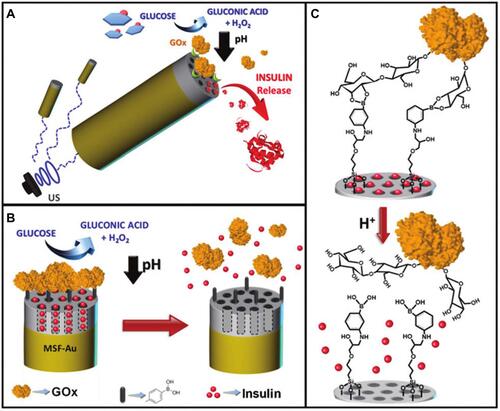