Figures & data
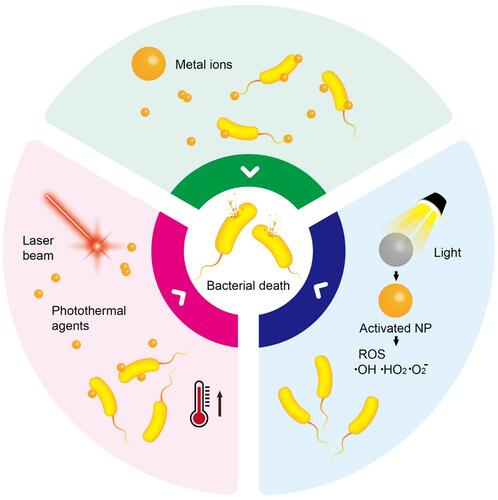
Figure 3 Schematic illustration of NPs dissolution, adsorption and hydrolysis. Reprinted from J Hazard Mater, 308, Wang D, Lin Z, Wang T, et al. Where does the toxicity of metaloxide nanoparticles come from: the nanoparticles, the ions, or a combination of both? 328–334, Copyright (2016), with permission from Elsevier.Citation90
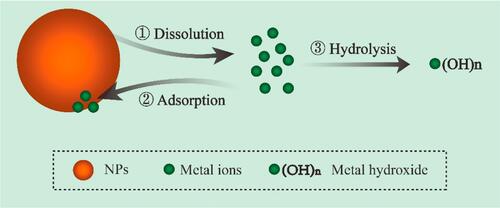
Figure 4 Schematic representation of several factors that can influence NPs behavior in solutions. Reprinted from Sci Total Environ, 438, Misra SK, Dybowska A, Berhanu D, et al. The complexity of nanoparticle dissolution and its importance in nanotoxicological studies. 225–232. Copyright 2012, with permission from Elsevier.Citation92
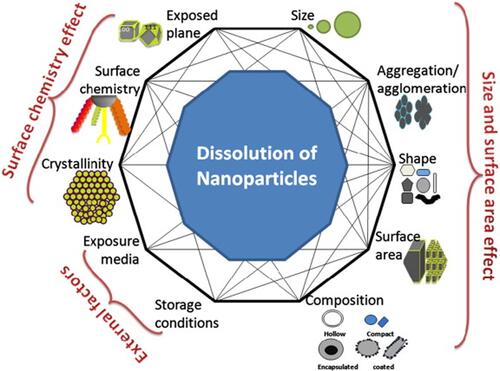
Figure 5 Schematic illustration of some NP characteristics capable of inducing ROS and subsequent bacteria death.
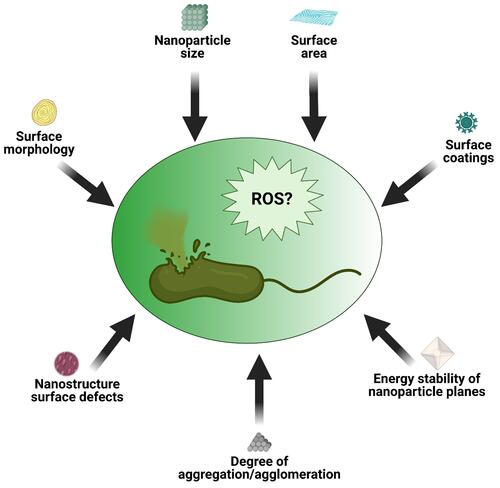
Figure 6 Schematic illustration of point of zero charge/NPs surface charge affects NPs bactericidal effects.
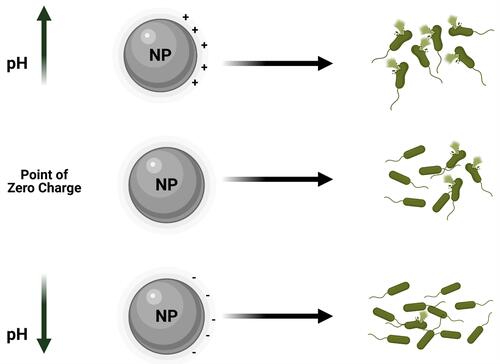
Table 1 Summary of NPs and Their Reported Mechanisms of Toxicity
Figure 8 Antibacterial mechanism of Ag/ZnO nanocomposites under light visible light irradiation. Reprinted from Catal Today, 339, Liu Q, Liu E, Li J, et al. Rapid ultrasonic-microwave assisted synthesis of spindle-like Ag/ZnO nanostructures and their enhanced visible-light photocatalytic and antibacterial activities. 391–402. Copyright 2020, with permission from Elsevier.Citation125
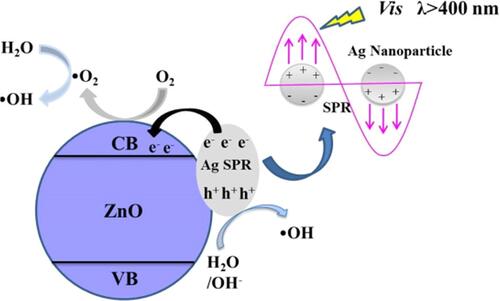
Figure 9 Antibacterial mechanism of TiO2-FeS2 nanocomposites under visible light irradiation by narrowing bandgap. Reproduced with permission from Dove Medical Press Limited. Mutalik C, Hsiao YC, Chang YH, et al. High uv-vis-nir light-induced antibacterial activity by heterostructured TiO2-FeS2 1470nanocomposites. Int J Nanomed. 2020;15:8911..Citation132
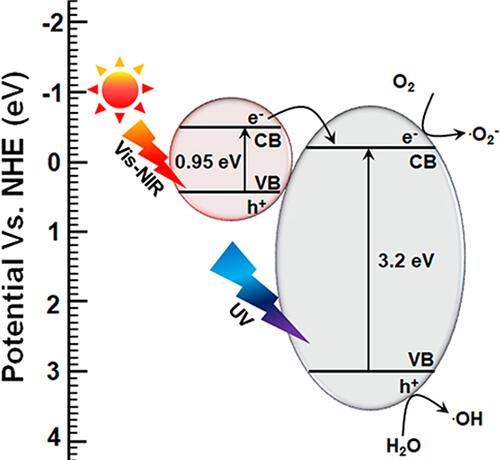
Figure 10 Antibacterial mechanism of Ag-ZnO-F3O4 nanocomposites under LED light irradiation. Reprinted from Appl Surf Sci. 527, Abutaha N, Hezam A, Almekhlafi FA, et al. Rational design of Ag-ZnO-Fe3O4 nanocomposite with promising antimicrobial activity under led light illumination. 146893, Copyright 2020, with permission from Elsevier.Citation139
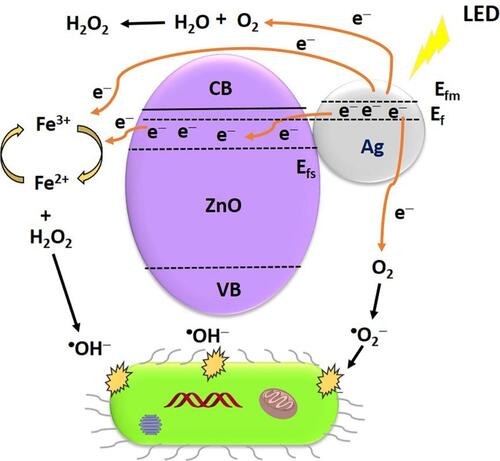
Figure 11 Schematic illustration of SPR effect from AgNPs under visible light irradiation (A), the Ag position in Ag@AgVO3/BiVO4 nanocomposites and performance under visible light irradiation (B), and Z-scheme arrangement of Ag in Ag@AgVO3/BiVO4 nanocomposites and performance under visible light irradiation (C). Reprinted from J Colloid Interface Sci. 579, Ju P, Wang Y, Sun Y, et al. In-situ green topotactic synthesis of a novel z-scheme Ag@agvo3/bivo4 heterostructure with highly enhanced visible-light photocatalytic activity. 431–447, Copyright 2020, with permission from Elsevier.Citation146
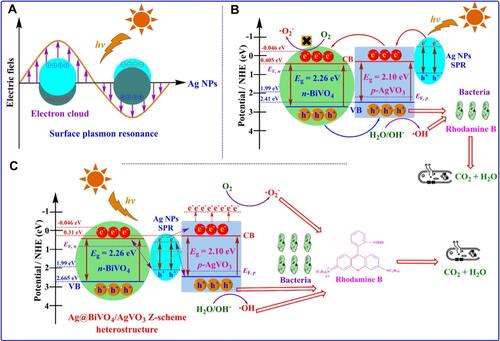
Figure 12 Schematic representation of antimicrobial mechanism of ZnO-Se nanocomposites under light irradiation. Reprinted from J Photochem Photobiol B, 203, Ahmad A, Ullah S, Ahmad W, et al. Zinc oxide-selenium heterojunction composite: synthesis, characterization and photo-induced antibacterial activity under visible light irradiation. 111743, Copyright 2020, with permission from Elsevier.Citation153
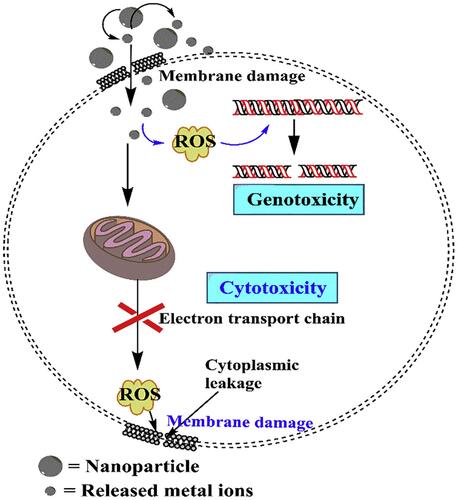
Figure 13 Schematic representation of antibacterial mechanism of g-C3N4/Cr-ZnO nanocomposites under simulated solar light irradiation. Reprinted from J Photochem Photobiol A, 401, Qamar MA, Shahid S, Javed M, et al. Highly efficient g-C3N4/cr-ZnO nanocomposites with superior photocatalytic and antibacterial activity. 112776, Copyright 2020, with permission from Elsevier.Citation158
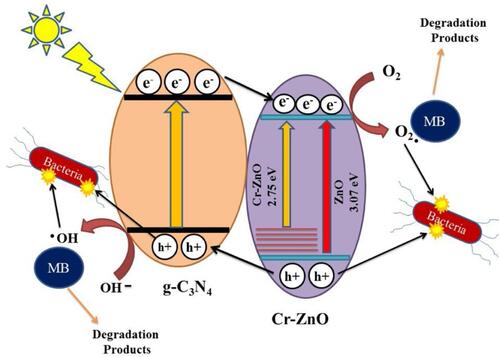
Figure 14 Schematic representation of the antibacterial mechanism of CuS/PCN composites under visible and laser light irradiation. Reprinted from J Hazard Mater. 393, Ding H, Han D, Han Y, et al. Visible light responsive CuS/protonated g-C3N4 heterostructure for rapid sterilization. 122423, Copyright 2020, with permission from Elsevier.Citation163
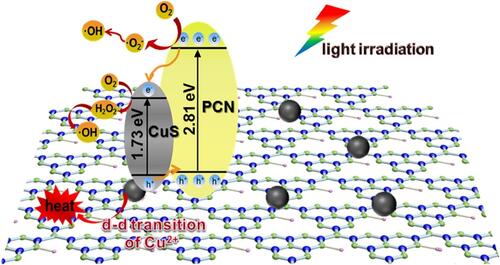
Figure 15 (A) Schematic representation of antibacterial mechanism of Fe3O4@SiO@Ag3PO4/ZnO-10% (Ag3PO4-10 wt%) (FSZA2-10%) microspheres under visible light irradiation and (B) bacterial cell wall damage by ROS generation. Reprinted from Colloids Surf, A Physicochem Eng Asp, 603, Mao K, Zhu Y, Zhang X, et al. Effective loading of well-dopedZnO/ag3po4 nanohybrids on magnetic core via one step for promotingits photocatalytic antibacterial activity. 125187, Copyright 2020, with permission with Elsevier.Citation168
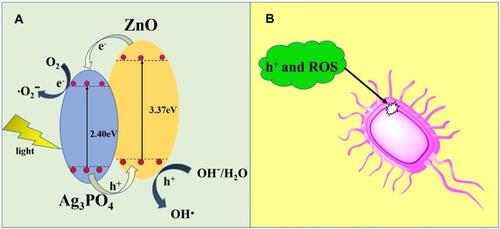
Table 2 Light-Driven Nanomaterials for Antibacterial Applications
Figure 16 Schematic representation of antibacterial mechanism of Ag/ Ag3PO4 combined MOF (MOF-5 or IRMOF-1) nanocomposites under visible light irradiation. Reproduced from Naimi Joubani M, Zanjanchi MA, Sohrabnezhad S. A novel Ag/ag3po4-irmof-1 nanocomposite for antibacterial application in the dark and under visible light irradiation. Appl Organomet Chem.2020;34:e5575. © 2020 John Wiley & Sons, Ltd.Citation173
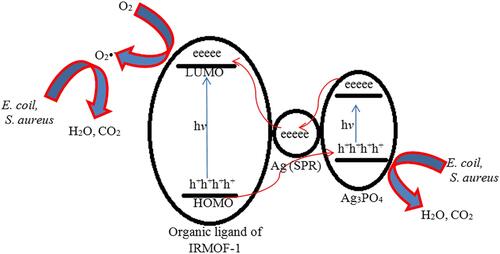
Figure 18 Schematic illustration of preparation and synergistic photothermal antibacterial activity of MoS2-PEI nanocomposite. Reprinted from J Photochem Photobiol A, 401, Cao W, Yue L, Khan IM, et al. Polyethylenimine modified MoS2nanocomposite with high stability and enhanced photothermal antibacterial activity. 112762, Copyright 2020, with permission from Elsevier.Citation201
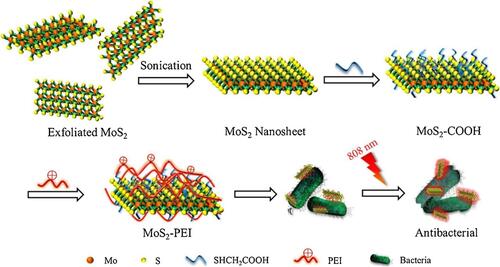
Figure 19 Photothermal bacterial selective killing therapy: (1) The charge conversion of Pep-DA/Au. (2) Bacterial targeting by nanocomposite. (3) Photothermal selective killing of bacteria.
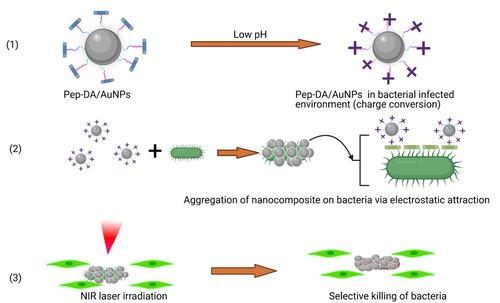
Figure 20 Synthesis of Caged Guanidine NPs and in vivo biofilm eradication via NIR irradiation. Reprinted with permission from Wang C, Zhao W, Cao B, et al. Biofilm-responsive polymeric nanoparticles with self-adaptive deep penetration for in vivo photothermal treatment of implant infection. Chem Mater.2020;32:7725–7738. Copyright (2020) American Chemical Society.Citation207
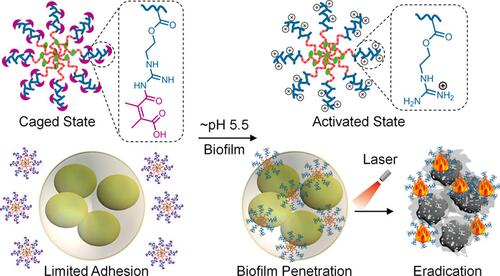
Figure 21 Schematic representation of specific bacterial recognition through Ab-PEG-GO-AuNPs via colorimetric detection and its photothermal ablation upon NIR irradiation. Reprinted from Sens Actuators B Chem, 329, Kaushal S, Pinnaka AK, Soni S, et al. Antibody assisted grapheneoxide coated gold nanoparticles for rapid bacterial detection and near infrared light enhanced antibacterial activity. 2021;329:129141, Copyright 2021, with permission from Elsevier.Citation210
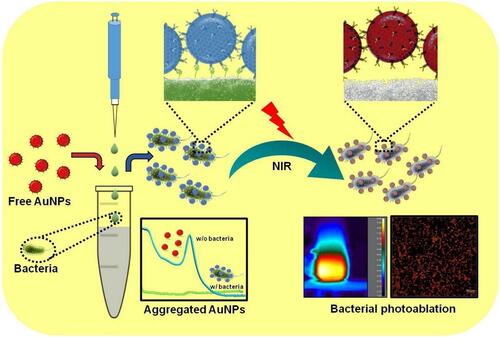
Figure 22 Diagram of RBCM-NW-G preparation and the treatment principle of bacterial infections in vivo. Reprinted from Bioact Mater, 6, Ran L, Lu B, Qiu H, et al. Erythrocyte membrane-camouflaged nanoworms with on-demand antibiotic release for eradicating biofilms using near-infrared irradiation. 2956–2968, Copyright 2021, with permission from KeAi Publishing.Citation216
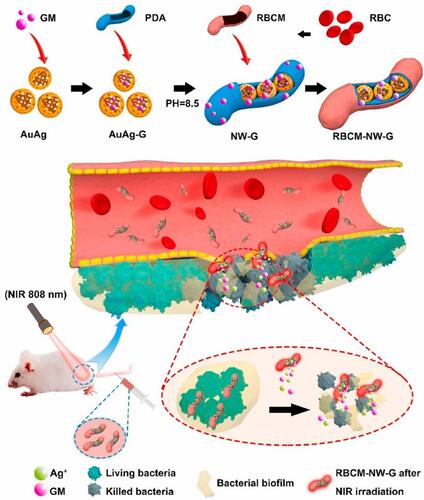
Figure 23 PPy-based photothermal nano-antibiotic (PTNA) for the treatment of multidrug-resistant bacterial infection. Reprinted with permission from Yang X, Xia P, Zhang Y, et al. Photothermal nano-antibiotic for effective treatment of multidrug-resistant bacterial infection. ACS Appl Bio Mater. 2020;3:5395–540. Copyright (2020). American Chemical Society.Citation217
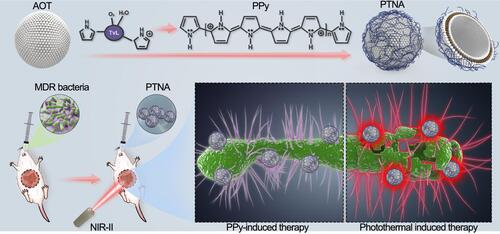
Figure 24 (A) Schematic illustration of stepwise synthesis of the final nano‐assembly. (B) Application of final nano‐assembly (GNR@MSNP@BDQ@TSL@NZX) for targeting and killing M. smegmatis using 808-nm NIR laser. Reprinted with permission from Patel U, Rathnayake K, Jani H, et al. Near-infrared responsive targeted drug delivery system that offer chemo-phototherma therapy against bacterial infection. Nano Select. 2021. © 2021 The Authors. Nano Select published by Wiley-VCH GmbH.Citation218
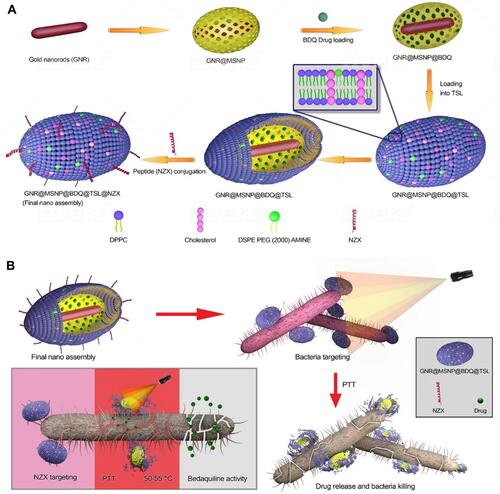
Figure 25 Design of BP/Au nanocomposite and its photothermal killing of bacteria. Reprinted with permission from Aksoy I, Kucukkececi H, Sevgi F, et al. Photothermal antibacterial and antibiofilm activity of black phosphorus/gold nanocomposites against pathogenic bacteria. ACS Appl Mater Interfaces. 2020;12:26822–26831. Copyright (2020), American Chemical Society.Citation223
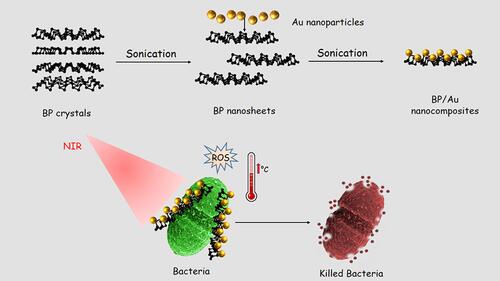
Figure 26 Schematic illustration of natural and photothermal antibacterial effect of AGO based on its positive charge, natural cutting effect, and photothermal property.
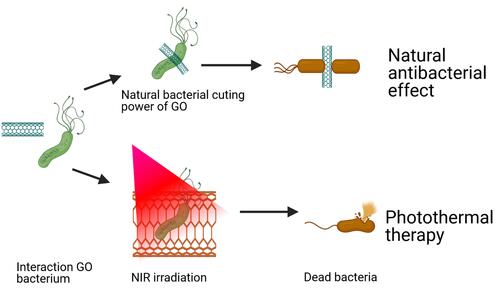
Table 3 Recap of Photothermal Bacterial Killing with Different Photothermal Agents (PTAs)
Figure 27 Schematic illustration of (A) the preparation of CS/AM NSs hydrogel and (B) its use in treating bacterial wound infection. Reproduced with permission from Liu Y, Xiao Y, Cao Y, et al. Construction of chitosan-based hydrogel incorporated with antimonene nanosheets for rapid capture and elimination of bacteria. Adv Funct Mater. 2020;30:2003196. © 2020 WILEY-VCH Verlag GmbH & Co. KGaA, Weinheim.Reproduced with permission from refCitation231
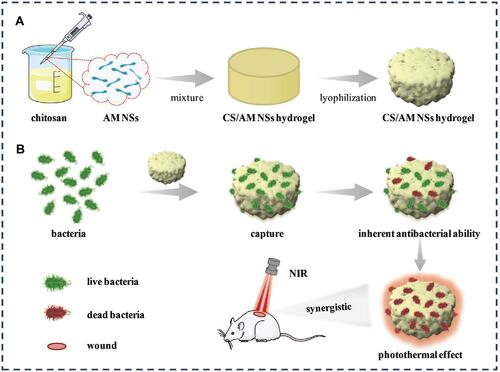