Figures & data
Figure 1 Synthesis and identification of TDN. (A) Four paired ssDNA molecules were assembled into a tetrahedral DNA nanostructure (TDN). (B) The molecular weights of ssDNA and TDN were determined using 8% PAGE, with the size of TDN estimated to be around 200 base pairs. The morphology of TDN was characterized using AFM (C) and TEM (D). The red circle indicated a pyramid-shaped structure of TDN, with a size of approximately 10–20 nm. Scale bar: 50 nm for the main figure and 20nm for detailed figure. (E) The size of TDN was analyzed using dynamic light scattering (DLS), which yielded an average size of 18 nm. (F) The zeta potential of the TDN exhibited a negative electric charge. (A–F) Reprinted with permission from Zhou M, Zhang T, Zhang B, et al. A DNA nanostructure-based neuroprotectant against neuronal apoptosis via inhibiting toll-like receptor 2 signaling pathway in acute ischemic stroke. ACS Nano. 2022,16,1,1456–1470. Copyright 2022 American Chemical Society.Citation46
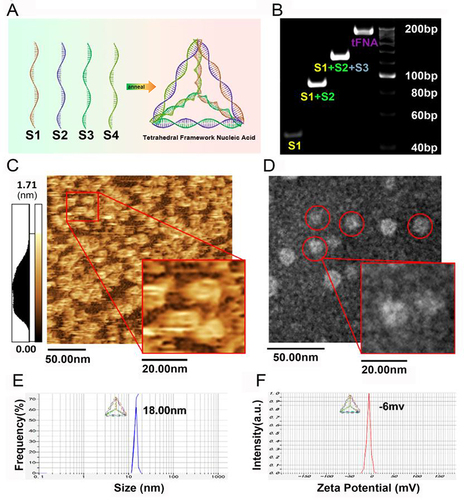
Figure 2 Enhanced cellular and tissue permeability. (A) Confocal images of intracellular internalization of Cy5-labeled naked TDN without the aid of transfection reagent. Blue: nuclear stain; red: Cy5; green: lysosomes; gray: phase contrast. Scale bars: 20 μm. Adapted with permission from from Walsh AS, Yin H, Erben CM, Wood MJA, Turberfield AJ. DNA Cage Delivery to Mammalian Cells. ACS Nano. 2011;5(7):5427–5432. Copyright 2021, with permission from American Chemical Society.Citation54 (B) Schematic illustration of 8 kinds of DNA nanostructures including Th21, Th37, Th337, 6H714, 6H14498, R13730, B14498, T14498. (C) Representative fluorescence images of mouse skin histology post various DNA nanostructures penetration (red: Cy5.5-labeled DNA nanostructures, blue: Hoechst 33342-stained skin). (D) Cy5.5 fluorescence signal from various DNA nanostructures along skin depth. (B–D) Reproduced from Wiraja C, Zhu Y, Lio DCS, et al. Framework nucleic acids as programmable carrier for transdermal drug delivery. Nat Commun. 2019;10(1):1147. Copyright 2019, with permission from Springer Nature.Citation26
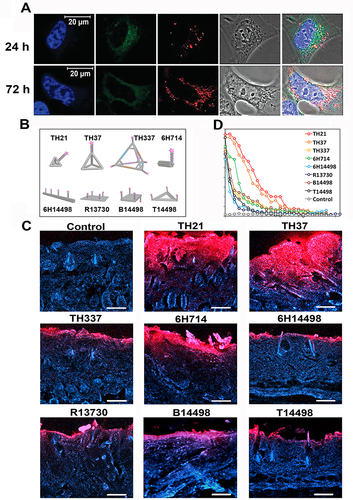
Figure 3 Schematic illustration of antibacterial applications of TDN-based delivery systems. Antibacterial agents, such as antibiotics, asPNA, AMPs, and ASOs, can be modified into TDN through intercalation and sticky-end hybridization methods. The resulting TDN-based nanoplatform is capable of inhibiting multiple bacterial strains through several mechanisms, including enhancing the uptake rate of antibiotics, preventing their efflux, promoting membrane destabilization, regulating bacterial transcription, and inhibiting biofilm formation.
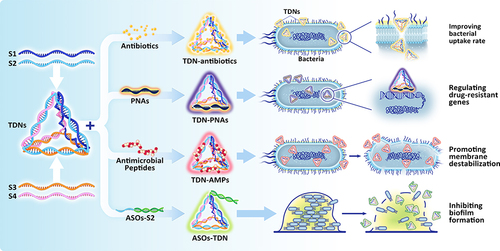
Figure 4 TDNs protect alveolar bone under inflammatory conditions. (A) Analysis of RUNX2 and OPN expression levels in PDLSCs exposed to TDNs and LPS using Western blotting, with statistical evaluation of results. Data are presented as means ± standard deviations (n = 3). ***P < 0.001. (B) Immunofluorescence staining imaging of OPN proteins. Scale bars are 50 μm. (C) Schematic diagram depicting the rat periodontitis experiment. (D) The images of H&E staining at 5×magnification and 20×magnification. The area surrounded by yellow solid line was cementum, and the area surrounded by yellow dotted line was the cementum absorbed by inflammation. The yellow arrow indicated the cementum in the yellow line. (E) The photographs of TRAP staining at 5×magnification and 20×magnification. The red arrow indicated the osteoclasts. The tFNA is equal to TDN. (D) dentin; (C) cementum. (A–E) Reproduced from Zhou M, Gao S, Zhang X, et al. The protective effect of tetrahedral framework nucleic acids on periodontium under inflammatory conditions. Bioactive Materials. 2021;6(6):1676–1688. Copyright 2020, with permission from Elsevier.Citation114
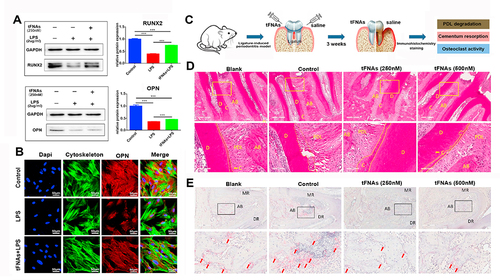
Figure 5 Using TDN to deliver osteogenic miRNA for bone regeneration. (A) Osteogenic differentiation was assessed by alkaline phosphatase detection and the statistical analysis results at day 5 are displayed. Scale bars are 200 μm. Data are presented as means ± standard deviations (n = 3). **P < 0.01. (B) Osteogenic differentiation at day 14 detected using alizarin red S staining and the statistical analysis results. Scale bars are 100 μm. Data are presented as means ± standard deviations (n = 3). **P < 0.01. (C) 3D reconstruction of the femur at day 14. (D) Representative images of Masson staining at 5×magnification. (E) The statistical analysis of the results of BV/TV, Tb.N, Tb.Sp, and Tb.Th (C). (F and G) The statistical analysis of collagen volume fraction at day 7 and day 14 (D). Data are presented as mean ± standard deviation (SD) (n = 5). *p < 0.05, **p < 0.01. (A–G) Reproduced from Li S, Liu Y, Tian T, et al. Bioswitchable delivery of microRNA by framework nucleic acids: application to bone regeneration. Small. 2021;17(47): e2104359. Copyright 2021, with permission from Wiley-VCH GmbH.Citation35
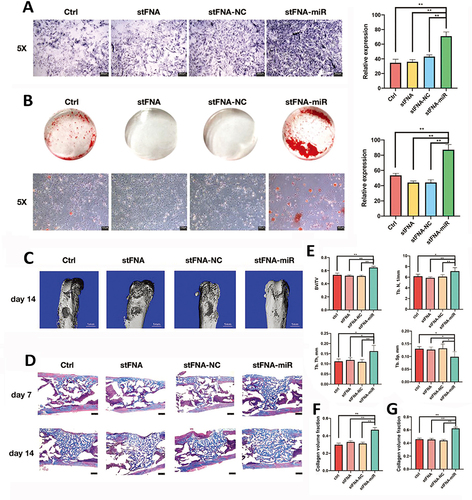
Figure 6 TDNs regulate chondrocyte performance. (A) Proliferation of chondrocytes detected by real-time cell analysis (RTCA). (B) The effect of TDNs on Chondrocyte migration through wound healing assays. (C) The statistical analysis of migrated cells. Data are presented as mean±SD (n=3). *P<0.05. (B and C) Reproduced from Shi S, Lin S, Shao X, Li Q, Tao Z, Lin Y. Modulation of chondrocyte motility by tetrahedral DNA nanostructures. Cell Prolif. 2017;50(5). Copyright 2017, with permission from John Wiley & Sons Ltd.Citation45 (D) The fluorescent images of cytoskeleton in chondrocytes. Scale bars are 25 µm. (E) Changes of cell area distribution after exposure to TDNs. (A, D and E) Reproduced from Shao X, Lin S, Peng Q, et al. Tetrahedral DNA nanostructure: a potential promoter for cartilage tissue regeneration via regulating chondrocyte phenotype and proliferation. Small. 2017;13(12). Copyright 2017, with permission from Wiley VCH.Citation19
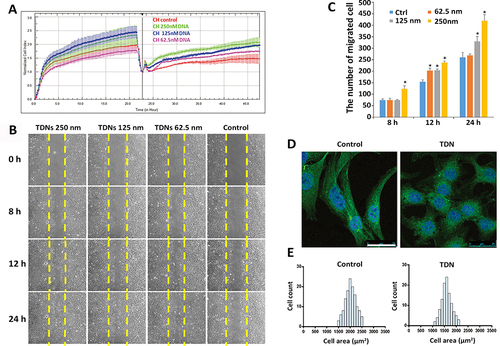
Figure 7 TDN-based strategy for cartilage repair and regeneration. (A) Schematic diagram of the effects of TDN/wogonin complex on osteoarthritis. The middle section showed the synthesis of TDN/wogonin complex. The upper section showed the TDN/wogonin complex alleviated the inflammation of chondrocytes in OA. The lower section showed the TDN/wogonin complex alleviated OA in the rat model. Reproduced from Sirong S, Yang C, Taoran T, et al. Effects of tetrahedral framework nucleic acid/wogonin complexes on osteoarthritis. Bone Res. 2020;8:6. Copyright 2020, with permission from Springer Nature.Citation25 (B) Schematic diagram of TDN-based strategy for articular repair and regeneration. In this study, a cartilage regenerative system was assembled based on a chitosan (CS) hydrogel/3D-printed poly(ε‐caprolactone) (PCL) hybrid containing synovial mesenchymal stem cells and recruiting Tetrahedral DNA nanostructure (TDN) injected into the articular cavity. Reproduced from Li P, Fu L, Liao Z, et al. Chitosan hydrogel/3D-printed poly(ε-caprolactone) hybrid scaffold containing synovial mesenchymal stem cells for cartilage regeneration based on tetrahedral framework nucleic acid recruitment. Biomaterials. 2021;278:121,131. Copyright 2021, with permission from Elsevier Ltd.Citation135
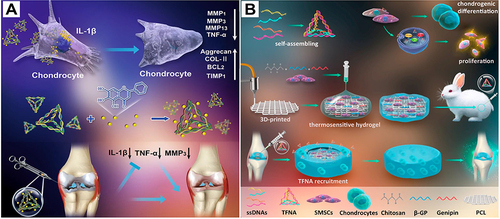