Figures & data
Figure 1 The impact of nanoparticle size, shape, and surface charge on interactions with macrophages. (A) Nanoparticle carriers quickly adsorb complement components from the plasma upon entering the vascular system, enhancing their recognition by macrophages. (B) Spherical particles of different sizes affect their interaction with macrophages. Particle sizes ranging from 30 to 3000 nm have been shown to increase macrophage recognition. Conversely, smaller nanoparticles below 30 nm or larger nanoparticles above 3 μm tend to reduce their interaction with macrophages. (C) Distinct nanoparticle shapes exhibit unique properties that significantly affect interaction with cell membranes, and uptake by macrophages. (D) The charge carried by nanoparticles, resulting from various surface chemistries, also affects their interaction with macrophages. Positively charged particles have a greater tendency to be captured by macrophages.
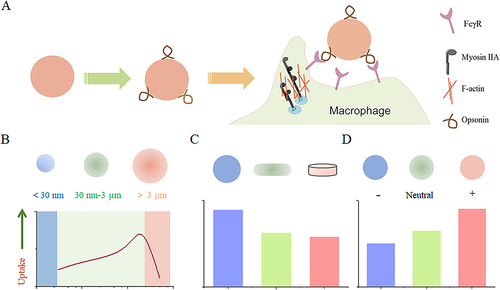
Table 1 Affinity Analysis Between Receptors and Ligands
Figure 3 Overview of commonly used carrier types: classified as organic or inorganic nanoparticles. Created with BioRender.com.
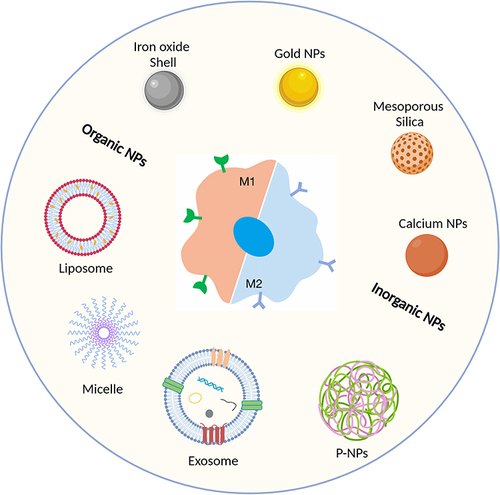
Table 2 Examples of Clinical Trials on Macrophage-Targeted Nanomedicine for Disease Treatment and Diagnosis
Figure 4 M1 and M2 macrophages have distinct roles in tumor therapy. M1 macrophages secrete pro-inflammatory cytokines like TNF-α, IL-6, and IL-12, which activate immune responses, enhance anti-tumor effects, and promote tumor cell apoptosis. In contrast, M2 macrophages release anti-inflammatory or immunosuppressive cytokines such as TGF-β, IL-10, IL-13, and IL-4, which create an immunosuppressive environment, inhibit anti-tumor responses, and contribute to angiogenesis and tissue remodeling. Therefore, activating M1 macrophages and inhibiting M2 macrophage activation can reduce tumor growth, metastasis, and angiogenesis.
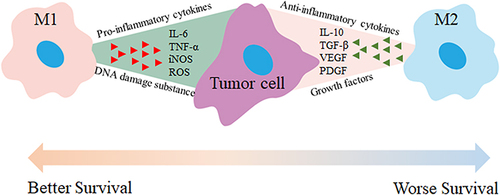
Figure 5 Enhancing precise recognition of M2 macrophages through combined Eat Me and Do not Eat Me signals. The interaction between the CD47 protein-derived Self peptide and the SIRPα protein on macrophage surfaces activates the Do not Eat Me signaling pathway, resulting in the inhibition of macrophage phagocytosis. Furthermore, the Do not Eat Me signal exhibits a tendency to suppress phagocytosis specifically in M1 macrophages. Conversely, the recognition of lactose by the highly expressed lactose receptor (MGL) on M2 macrophages triggers the Eat Me signal. The recognition between receptors and ligands shows selective range specificity, wherein the interaction between lactose and MGL indirectly creates spatial hindrance against the Self peptide-SIRPα protein interaction, consequently inhibiting the response of M2 macrophages to the Do not Eat Me signal. Reprinted from Tang Y, Tang Z, Li P et al. Precise delivery of nanomedicines to M2 macrophages by combining “eat me/don’t eat me” signals and its anticancer application. ACS Nano. 2021; 15(11): 18,100–18,112. Copyright 2021, with permission from ACS publications.Citation105
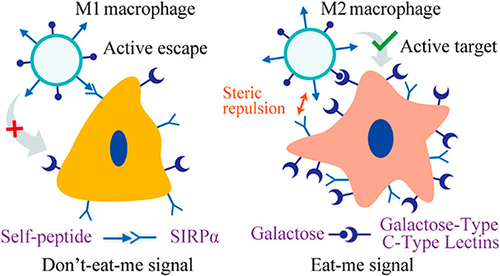
Figure 6 (A) Proposed mechanism of dextran-dexamethasone conjugate accumulation in obese Visceral Adipose Tissue (VAT), macrophage uptake, and uncoupling of the paracrine loop between M1 macrophages and adipocytes: (a) In obese mice following intraperitoneal left-side injection, dextran conjugates (highlighted in green) accumulate in the left perirenal adipose tissue (AT) and left gonadal AT. The anatomical depiction shows mice with different fat depots: one mesenteric, two perirenal, and two gonadal AT depots. (b) A transverse cross-section of the mouse abdomen illustrates the localization of the green dextran solution after administration into the peritoneal cavity. (cInflamed adipose tissue enables rapid association of dextran conjugates with M1 macrophages by transport across the peritoneum to directly access interstitial cells. (B) Dextran nanocarrier uptake by myeloid cells in the adipose tissue of obese mice. Obese mice were intraperitoneally injected with D-70-fluor. Twenty-four hours later, the left gonadal adipose tissue was isolated, sectioned, and stained with Hoechst dye. Confocal laser scanning microscopy images show the Hoechst channel (top left), the D-fluor channel (top right), bright-field microscopy (bottom left), and the overlay (bottom right). D-70 was observed within the interstitial space between adipocytes. Scale bars: 200 μm. (C) In vivo anti-inflammatory effects of D-70-drug in obese mouse VAT. Representative hematoxylin-and-eosin-stained images of paraffin-embedded gonadal adipose tissue isolated from each mouse group. Scale bar: 100 μm. Reprinted from Ma L, Liu TW, Wallig MA et al. Efficient targeting of adipose tissue macrophages in obesity with polysaccharide nanocarriers. ACS Nano. 2016;10(7): 6952–6962. Copyright 2016, with permission from ACS publications.Citation171
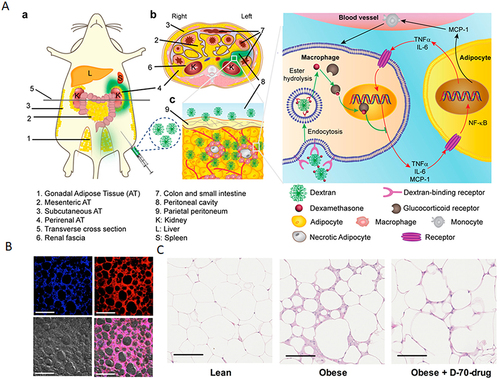
Figure 7 PLGA nanoparticle-based delivery of SYK pathway inhibitors for NASH treatment. This study utilized R406, a small molecule inhibitor, and synthesized PLGA nanoparticles to block the Fc receptor signaling pathway, specifically inhibiting the SYK pathway and reducing immune complex-mediated inflammation. Reprinted from Kurniawan DW, Jajoriya AK, Dhawan G et al. Therapeutic inhibition of spleen tyrosine kinase in inflammatory macrophages using PLGA nanoparticles for the treatment of non-alcoholic steatohepatitis. J Control Release. 2018; 288: 227–238. Creative Commons.Citation180
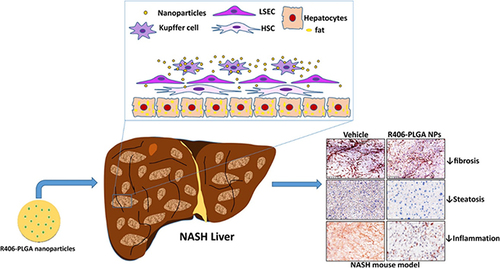
Figure 8 Engaging molecularly engineered M2 macrophage-derived exosomes with inflammation tropism and anti-inflammatory capabilities for co-delivery of IL-10 pDNA and GCs to achieve rheumatoid arthritis (RA) treatment via M1-to-M2 macrophage repolarization. Reprinted from J Control Release, 341, Li H, Feng Y, Zheng X et al. M2-type exosomes nanoparticles for rheumatoid arthritis therapy via macrophage re-polarization. 16–30. Copyright 2022, with permission from Elsevier.Citation195
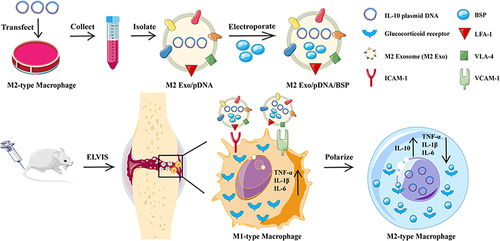
Figure 9 Schematic illustration of the combinational regulation of plaque microenvironment by plaque macrophage-targeted nanosystems: 37pALNP/6877002 and 37pA-PtLNP. During atherosclerosis (AS) therapy, 37pA-PtLNP effectively scavenges intracellular reactive oxygen species (ROS) in inflammatory macrophages within the plaque. On the other hand, 37pA-LNP/6877002 inhibits the activities of TRAF6, leading to a modest decrease in the proportion of the proinflammatory macrophage phenotype (reducing the expression of CD86 and iNOS). More importantly, the combination of 37pA-PtLNP and 37pA-LNP/6877002 regulates the atherosclerotic plaque microenvironment and achieves satisfactory stabilization of plaques with minimal progression. Reprinted from Yang Q, Jiang H, Wang Y et al. Plaque Macrophage-Targeting Nanosystems with Cooperative Co-Regulation of ROS and TRAF6 for Stabilization of Atherosclerotic Plaques. Adv. Funct. Mater. 2018;33: 2,301,053. © 2023 Wiley-VCH GmbH.Citation201
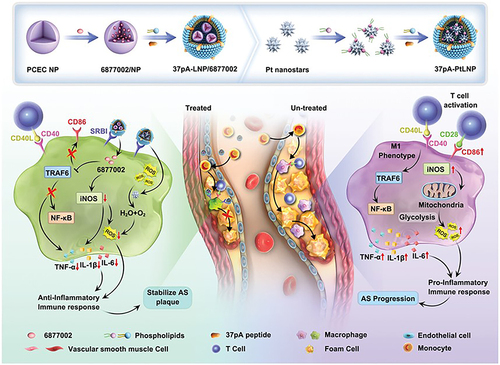
Figure 10 The microspheric vehicle, 3# KPM, composed of cationic konjac glucomannan (cKGM), phytagel, and an antisense oligonucleotide against TNF-α, showed anti-colitis activity. (A) Body weight changes were observed over time. (B) Survival analysis. (C) Disease activity index was calculated to evaluate the severity of colitis. (D) myeloperoxidase (MPO) activity determination. (E and F) Colon length was measured as an indicator of inflammation and tissue damage. (G and H) Colon sections were stained with H&E and histopathological scoring was conducted to assess inflammatory cell infiltration and tissue damage. Scale bar: 100 μm. (I) The levels of colonic inflammatory cytokines (IL-1β, IL-6, IL-12p70, and IL-23) were determined in DSS colitic mice with different treatments. Results are expressed as the mean (SEM). *p<0.05. Reprinted from Biomaterials; 48, Huang Z, Gan J, Jia L et al. An orally administrated nucleotide-delivery vehicle targeting colonic macrophages for the treatment of inflammatory bowel disease. 26–36. Copyright 2015, with permission from Elsevier.Citation215
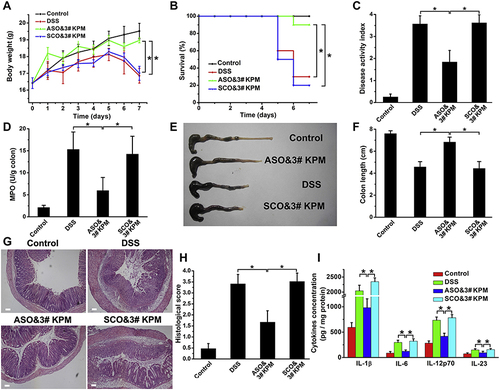