Figures & data
Figure 1 Inhalation of nanoparticles into the lungs. (A) Comparison of the relative sizes of nanomaterials with microbiological and other biological entities. Reprinted from Poh TY, Ali N, Mac Aogáin M, et al Inhaled nanomaterials and the respiratory microbiome: clinical, immunological and toxicological perspectives. Particle and fibre toxicology. 2018;15(1):46.Citation22 (B) Diagrammatic illustration of the respiratory tract and physiological factors. Reprinted from Advanced Drug Delivery Reviews, 185, Wang W, Huang Z, Huang Y, et al, Pulmonary delivery nanomedicines towards circumventing physiological barriers: strategies and characterization approaches, 114309, Copyright 2022, with permission from Elsevier.Citation23
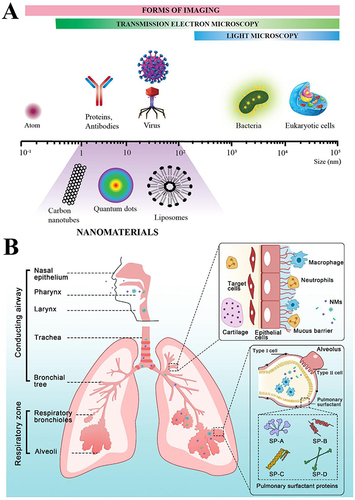
Figure 2 (A) Substitution of auxiliary lipids by charged alternatives in lipid nanoparticles enables the specific delivery of mRNA to the spleen and lungs. (i) Structure of three auxiliary lipids. (ii) Helper lipid charge affects the organ location of protein expression after mRNA delivery. (iii) Detection of helper lipid properties affecting the inflammatory state and the cell-specific location of protein expression in organs using firefly luciferase (green), globin-like enzyme (red), and Hoechst (blue) antibody staining.Citation32 Adapted from LoPresti ST, Arral ML, Chaudhary N, Whitehead KA. The replacement of helper lipids with charged alternatives in lipid nanoparticles facilitates targeted mRNA delivery to the spleen and lungs. J Control Release. 2022;345:819–831.Citation32 (B) (i) Schematic demonstration of the fate of nanoparticles inhaled into the alveoli. (ii) Descriptive cryo-TEM images of multilamellar vesicles (MLVs) of PS, LPNs, and LPNs/PS combinations. (a) Non-modified PLGA nanoparticles. (b) non-modified PLGA nanoparticles with MLVs of PS. (c) 15% (w/w) L5N12-modified LPNs. (d) 50% (w/w) L5N12-modified LPNs. (e) 15% (w/w) L5N12-modified LPNs with MLVs of PS. (f) 50% (w/w) L5N12-modified LPNs with MLVs of PS. Red arrows refer to the interaction area between LPNs and PS.Citation14 PS, pulmonary surfactant; LPN, liposomal polymer nanoparticles; Adapted from Xu Y, Parra-Ortiz E, Wan F, et al. Insights into the mechanisms of interaction between inhalable lipid-polymer hybrid nanoparticles and pulmonary surfactant. J Colloid Interface Sci. 2023;633:511–525.Citation14
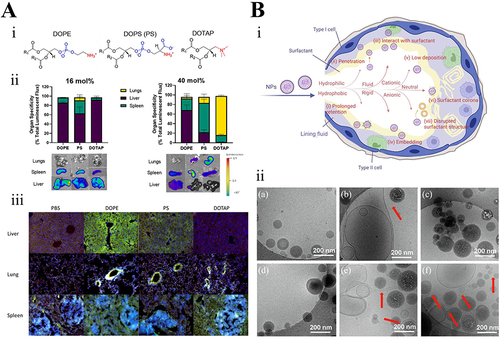
Figure 3 Deposition of particulate matter in the respiratory system. (A) Inhaled particles: accumulation mechanisms occurring through the respiratory tract.Citation71 Reprinted from Bessa MJ, Brandão F, Rosário F, et al. Assessing the in vitro toxicity of airborne (nano) particles to the human respiratory system: from basic to advanced models. J Toxicol Environ Health B Crit Rev. 2023;26(2):67–96.Citation71 (B) The respiratory tract of individuals at rest in relation to particle deposition and particle size.Citation72 Reprinted from Geiser M, Kreyling WG. Deposition and biokinetics of inhaled nanoparticles. Part Fibre Toxicol. 2010;7:2.Citation72
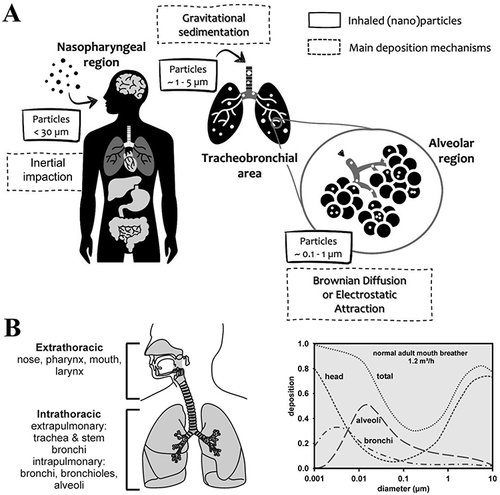
Figure 4 Nanoparticles in lung tumors. (A) Smart transformable nanomedicines with a schematic representation for overcoming biological barriers and enhancing anti-tumor efficacy. Reprinted from Biomaterials, 271, Wang Y, Li S, Wang X, et al, Smart transformable nanomedicines for cancer therapy, 120737, Copyright 2021, with permission from Elsevier.Citation108 (B) Synthesis and mechanism of action of SPIO@PSS/CDDP/HSA-MTX NPs.Citation111 Reprinted from Yang SJ, Huang CH, Wang CH, Shieh MJ, Chen KC. The Synergistic Effect of Hyperthermia and Chemotherapy in Magnetite Nanomedicine-Based Lung Cancer Treatment. Int J Nanomedicine. 2020;15:10331–10347.Citation111
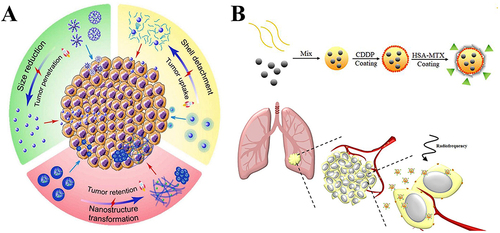
Figure 5 Schematic of the multi-modular peptide-based gene transfection platform. (A) Structures of the synthetic multi-modular peptide and poloxamine 704 (T704). (B) Schematic exhibiting nanoparticle formation. (C) A suggested pulmonary delivery mechanism of pDNA-BC, pDNA-TC, and mRNA-TC.Citation112 Reprinted with permission from Guan S, Munder A, Hedtfeld S, et al. Self-assembled peptide-poloxamine nanoparticles enable in vitro and in vivo genome restoration for cystic fibrosis. Nat Nanotechnol. 2019;14(3):287–297. doi:10.1038/s41565-018-0358-x. Copyright 2019 Springer Nature.Citation112
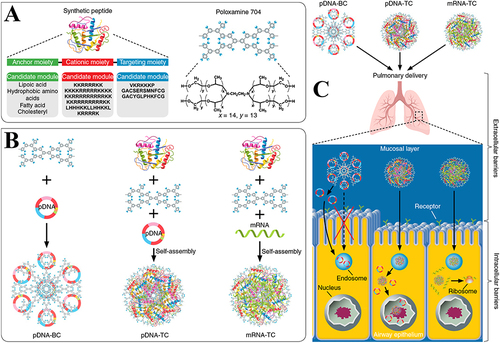
Figure 6 Nanotechnology applied to the detection and treatment of asthma. (A) The nanomaterial-based selective sensing technique against the nanomaterial-based cross-reactive sensing technique. Used with permission of Future Medicine Ltd, from Nanomaterial-based sensors for detection of disease by volatile organic compounds, Broza YY, Haick H, 8, 5, copyright 2013; permission conveyed through Copyright Clearance Center, Inc.Citation123 (B) The synthetic pathway of PHEA-g-RhB-g-SUCCPCL-g-PEG graft copolymer.Citation126 Copyright 2022, reprinted with permission from Springer.Citation126 (C) Muco-diffusion test of Rapa-loaded nanoPEG by a mucus layer.Citation126 Reprinted from Craparo EF, Drago SE, Quaglia F, Ungaro F, Cavallaro G. Development of a novel rapamycin loaded nano- into micro-formulation for treatment of lung inflammation. Drug Deliv Transl Res. 2022;12(8):1859–1872.Citation126
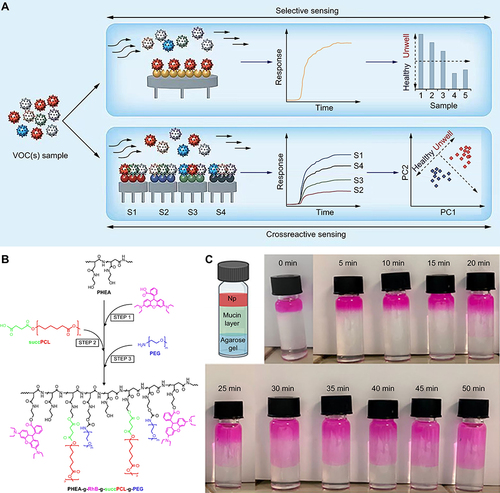
Figure 7 Nanoparticles applied to the treatment of lung infections. (A) (i) Chemical structure of PLA NP constituents (nanoemulsion technique) and description of the mean diameter of NPs utilizing three different techniques: PCS (number average data), NTA, and cryo-TEM. (ii) Imaging analysis demonstrated the intracellular anti-tuberculosis activity.Citation132 Reprinted from Costa-Gouveia J, Pancani E, Jouny S, et al. Combination therapy for tuberculosis treatment: pulmonary administration of ethionamide and booster co-loaded nanoparticles. Sci Rep. 2017;7(1):5390.Citation132 (B) Biological barriers within pulmonary drug delivery. Targeted liposomes and liposomal dry powder formulations loaded with bedaquiline (BDQ) and levofloxacin (LVX) sequentially underwent construction to address these barriers.Citation133 Reprinted from Huck BC, Thiyagarajan D, Bali A, et al. Nano-in-Microparticles for Aerosol Delivery of Antibiotic-Loaded, Fucose-Derivatized, and Macrophage-Targeted Liposomes to Combat Mycobacterial Infections: In Vitro Deposition, Pulmonary Barrier Interactions, and Targeted Delivery. Adv Healthc Mater. 2022;11(11):e2102117.Citation133
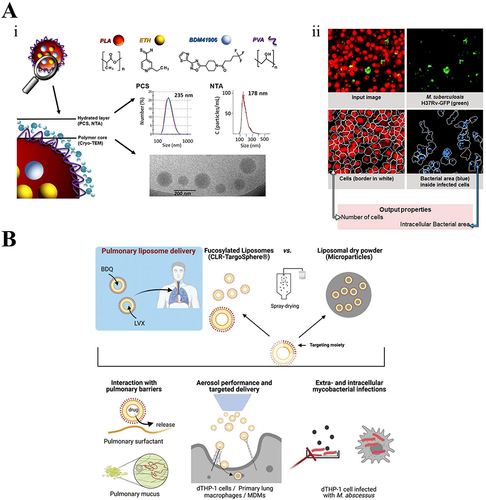
Figure 8 Nanovaccines for the prevention and treatment of respiratory tumors. (A) Customizing patient-specific cancer vaccines. From Sahin U, Türeci Ö. Personalized vaccines for cancer immunotherapy. Science. 2018;359(6382):1355–1360. doi:10.1126/science.aar7112. reprinted with permission from American Association for the Advancement of Science (AAAS).Citation140 (B) Description of a MnOx-OVA/tumor cell fragment. Reprinted with permission from Ding B, Zheng P, Jiang F, et al. MnO(x) nanospikes as nanoadjuvants and immunogenic cell death drugs with enhanced antitumor immunity and antimetastatic effect. Angewan Chem. 2020;59(38):16381–16384. doi:10.1002/anie.202005111. © 2020 Wiley-VCH Verlag GmbH & Co. KGaA, Weinheim.Citation146 (C) MnOx nanospikes against tumors. (i) H&E, TUNEL, CD8, and CRT stainings to assess treatment efficacy. (ii) H&E staining of lungs within antimetastatic investigations. The red arrows represent the metastatic nodules. Reproduced with permission from Ding B, Zheng P, Jiang F, et al. MnO(x) nanospikes as nanoadjuvants and immunogenic cell death drugs with enhanced antitumor immunity and antimetastatic effect. Angewan Chem. 2020;59(38):16381–16384. doi:10.1002/anie.202005111. © 2020 Wiley-VCH Verlag GmbH & Co. KGaA, WeinheimCitation146 (D) SVMAV decreased the pulmonary metastasis of melanoma. (i) Representative H&E staining and Ki-67 immunohistochemical staining findings of different groups of lung sections. (ii) Photograph of resected lung tissue on day 37; red arrows indicate metastases.Citation147 Reprinted fromZhang L, Huang J, Chen X, et al. Self-assembly nanovaccine containing TLR7/8 agonist and STAT3 inhibitor enhances tumor immunotherapy by augmenting tumor-specific immune response. J Immunother Cancer. 2021;9(8):e003132.Citation147
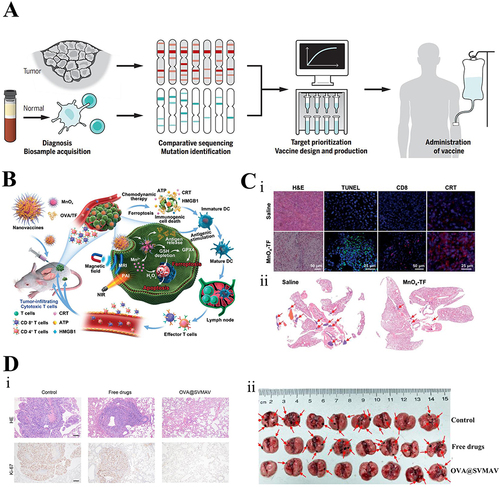
Figure 9 Nano virus vaccines in the respiratory system. (A) The antigenic cargo undergoes processing through the APC, and epitopes are introduced via MHC-I and MHC-II, resulting in the synthesis of CD8+ cytotoxic T cells or CD4+ T helper cells needed for antiviral antibody synthesis. Reprinted with permission from Shin MD, Shukla S, Chung YH, et al. COVID-19 vaccine development and a potential nanomaterial path forward. Nature Nanotechnol. 2020;15(8):646–655. doi:10.1038/s41565-020-0737-y. Copyright 2020, Springer Nature.Citation150 (B) Major delivery methods for mRNA vaccines. Copyright 2018, reprinted with permission from Springer Nature.Citation154 (C) Structural modeling of SARS-CoV-2, SARS, and MERS spiny glycoproteins, based on which a targeted DNA nanovaccine was designed (antigen).Citation155 Reprinted from Smith TRF, Patel A, Ramos S, et al. Immunogenicity of a DNA vaccine candidate for COVID-19. Nat Commun. 2020;11(1):2601.Citation155
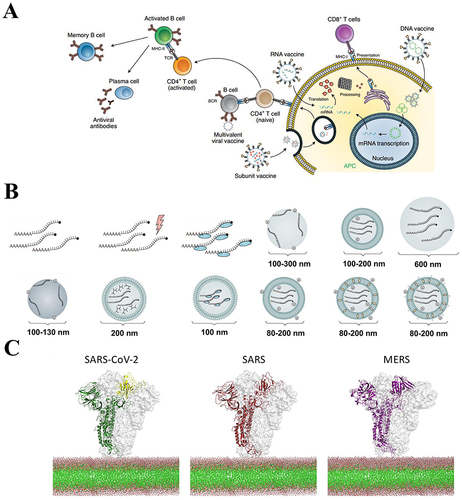
Figure 10 (A) Overlayed 1H (gray) and 19F (red) images of rabbit VX-2 orthotopic lung cancer models. Representative images prior to and at 0.5, 2, 3, 5, and 24 h following intratracheal (i) or intravenous (ii) administration of ανβ3-targeted PFC nanoparticles. Used with permission of Future Medicine Ltd, from (19)F MRI in orthotopic cancer model via intratracheal administration of α(ν)β(3)-targeted perfluorocarbon nanoparticles, Xu X, Zhang R, Liu F, et al, 13, 20, copyright 2018; permission conveyed through Copyright Clearance Center, Inc.Citation162 (B) Magnetic particle imaging (MPI) can evaluate the delivery efficacy of various techniques. (i) Principle of operation of MPI. (ii) MPI evaluation of the delivery efficacy of three distinct techniques.Citation164 Reprinted from Tay ZW, Chandrasekharan P, Zhou XY, Yu E, Zheng B, Conolly S. In vivo tracking and quantification of inhaled aerosol using magnetic particle imaging towards inhaled therapeutic monitoring. Theranostics. 2018;8(13):3676–3687.Citation164
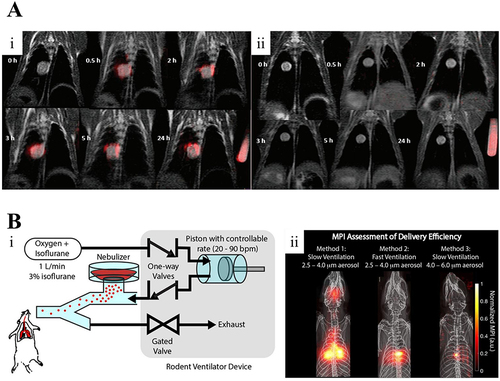