Figures & data
Table 1 Monoamine Uptake Inhibition, Transporter Binding Affinity, and Selectivity for Viloxazine, Atomoxetine, and Reboxetine.
Figure 1 Neurotransmitter transporters and receptors binding profile of viloxazine. Representative results of binding competition assays of viloxazine (10 µM). Viloxazine binding was calculated as a percent inhibition of the binding of a radiolabeled ligand specific for each target (mean ± SEM, n = 2). Targets that presented an inhibition higher than 50% were considered a significant effect for viloxazine binding.
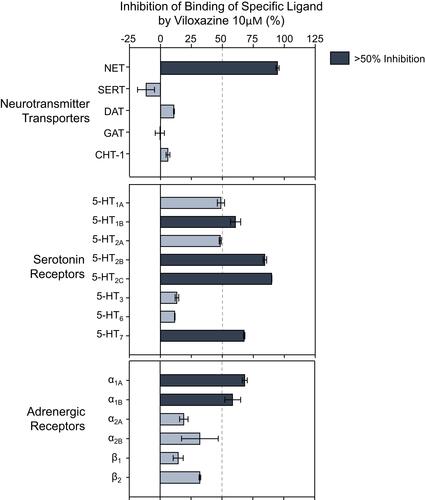
Figure 2 Effects of viloxazine on uptake and cellular functional activity in vitro. (A) Dose-dependent inhibition of NET-mediated [3H]-NE uptake (n = 3) measured in rat hypothalamic synaptosomes and [3H]-5-HT uptake (n = 3) measured in HEK293 cells expressing hSERT. (B) Viloxazine activated the response of 5-HT2C (agonist) in a CHO cell-based IP1 HTRF® assay (n = 2). (C) Viloxazine antagonized the activity of 5-HT2B after stimulation with 5-HT in a CHO cell-based IP1 HTRF® assay (n = 2). Data presented as mean ± SEM.
![Figure 2 Effects of viloxazine on uptake and cellular functional activity in vitro. (A) Dose-dependent inhibition of NET-mediated [3H]-NE uptake (n = 3) measured in rat hypothalamic synaptosomes and [3H]-5-HT uptake (n = 3) measured in HEK293 cells expressing hSERT. (B) Viloxazine activated the response of 5-HT2C (agonist) in a CHO cell-based IP1 HTRF® assay (n = 2). (C) Viloxazine antagonized the activity of 5-HT2B after stimulation with 5-HT in a CHO cell-based IP1 HTRF® assay (n = 2). Data presented as mean ± SEM.](/cms/asset/d3bf2f34-c9fa-449c-b15a-48bac714a9c1/djep_a_12167284_f0002_b.jpg)
Table 2 Statistical Analysis of the Effects of Viloxazine Administration on Monoamine Levels in the Three Brain Regions as Measured by Microdialysis in Freely Moving Rats
Figure 3 Effects of viloxazine on neurotransmitter levels in the PFC, Acb, and Amg of freely moving rats. Administration of viloxazine (50 mg/kg, IP) at time 0 is indicated by the arrow. (A) In the PFC, viloxazine significantly increased extracellular levels of NE and DA throughout the 4 h period (**p < 0.001, Dunnett’s post hoc test) and 5-HT from 30 to 120 minutes (#p < 0.05 Dunnett’s post hoc test) in comparison to vehicle treated groups. (B) In the Acb, extracellular levels of 5-HT and NE increased throughout the 4 h period (**p < 0.001, Dunnett’s post hoc test) and DA from 30 to 60 min (#p < 0.05, Dunnett’s post hoc test). (C) In the Amg, extracellular levels of 5-HT, NE, and DA increased throughout the 4 h period (**p < 0.001, Dunnett’s post hoc test). Measured neurotransmitter levels (mean ± SEM) are reported as the percent of pre-treatment baseline. The statistical post-hoc analyses of vehicle vs viloxazine at each time point (T = 0 to T = 240) and significant interactions (two-way ANOVA with p<0.05) are presented in the .
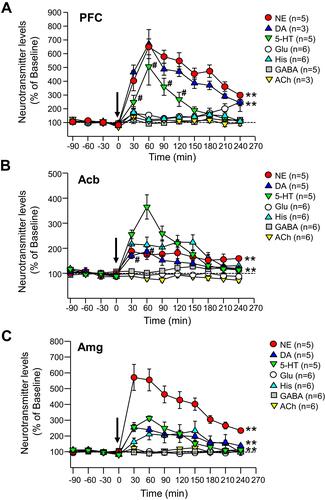
Table 3 Receptor Occupancy Calculated Based on Obtained Cunbound Brain Concentration Estimated from Cmax Plasma After 100, 200, 400 and 600 mg/day Doses in Pediatric ADHD Patients and Ki Values of 630, 3900 and 6400 nM for NET, 5-HT2B and 5-HT2C, Respectively
Figure 4 Dose – Calculated Receptor Occupancy Curves. Relationship between the dose of administered viloxazine to pediatric ADHD patients (31.5 kg mean body weight) and the estimated receptor occupancy for NET, 5-HT2B, and 5-HT2C based on the estimated unbound brain concentration of viloxazine at target receptor and its binding affinity (Ki) at clinical doses of 100, 200, 400, and 600 mg/day.
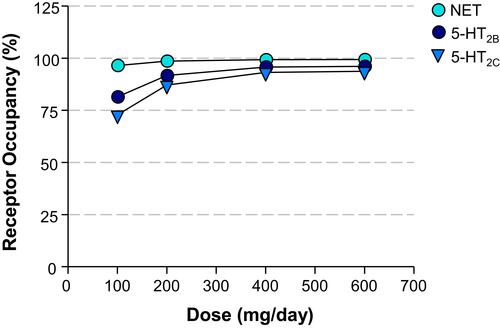
Figure 5 Complex regulation of 5-HT projections to prefrontal cortex. 1. “Proximal” regulatory loop includes feedback collateral branches and 5-HT1A autoreceptors, whose activation by excessive neurotransmitter results in reduced 5-HT synthesis and neuronal firing rate. Feedback collaterals also synapse with 5-HT2B receptors on DNR GABA interneurons. Inhibition of these receptors may be one of the principal regulators of 5-HT tonic neurotransmission. Viloxazine, for instance, may inhibit 5-HT2B receptors leading to inhibition of GABAergic transmission and consequently to upregulation of 5-HT tonic neurotransmission in mPFC, which may explain the increase of 5-HT in PFC observed in current microdialysis study. 2. At the terminal end 5-HT1B/1D autoreceptors regulate the 5-HT release. Serotoninergic projections in mPFC interface with SST-expressing and PV-expressing GABA interneurons as well as directly modulate glutamatergic pyramidal neurons via postsynaptic inhibitory 5-HT1A receptors and excitatory 5-HT2A receptors. SST GABA interneurons have a primary role in “signal-to-noise” gating of pyramidal neurons, while PV interneurons via axonal receptors regulate “volume” of glutamate transmission. 3. Serotoninergic input, indirectly, via mPFC GABA interneurons, and directly, via pyramidal neurons, regulates initiation of the “distal” regulatory loop. Additionally, the balance between activation of 5-HT2C receptors on GABA interneurons vs direct stimulation of 5-HT2C receptors located on pyramidal neurons, ultimately tunes glutamatergic output from mPFC. 4. Glutamatergic projections from mPFC to LC noradrenergic neurons, VTA dopaminergic neurons, and DNR/MNR serotoninergic neurons, as well as their corresponding GABA interneurons, directly and indirectly regulate function of the brainstem monoaminergic nuclei. Local balance between stimulation of 5-HT2C receptors on GABA interneurons vs ones located directly on VTA DA neurons, modulates DA output from the nucleus. At the termination point of the “distal” loop, glutamate fibers via excitatory AMPA and NMDA receptors located on 5-HT neurons and adjacent GABA interneurons, provide balanced regulatory input to serotoninergic nuclei.
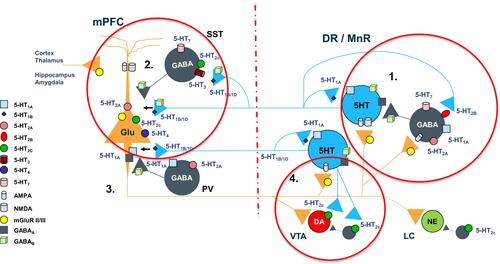
Figure 6 Proposed dual mechanism of action of viloxazine. The schematic shows purported clinical implications (dashed lines) of the observed neurotransmitter modulating effects of viloxazine. Microdialysis and in vitro studies demonstrate that viloxazine increases 5-HT, NE, and DA levels in the PFC of awake rats, as well as exhibits antagonistic activity towards 5-HT2B receptors and agonistic activity towards 5-HT2C receptors. These effects of viloxazine on modulating both the 5-HT and NE systems help explain why viloxazine is therapeutically relevant to neuropsychiatric disorders, such as the core symptoms of ADHD and depression.
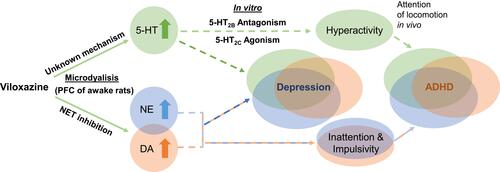