Figures & data
Table 1 Potential Of DNA Nanostructures In Biomedicine
Figure 1 Concept of DNA tile and DNA origami assembly methods. (A) Tile method for mounting DNA grids. Reprinted with permission from Manuguerra I, Grossi G, Thomsen RP, et al. Construction of a polyhedral DNA 12-arm junction for self-assembly of wireframe DNA lattices. ACS Nano. 2017;11(9):9041–9047. doi:10.1021/ acsnano.7b03538.Citation165 Copyright (2017) American Chemical Society. (B) a, Principle design of DNA origami. Adapted by permission from Springer Nature: Nature, Rothemund PWK. Folding DNA to create nanoscale shapes and patterns. 2006;440:297. doi:10.1038/nature04586.Citation166 Copyright (2006). b,d, DNA origami objects. b, From Han D, Pal S, Nangreave J, Deng Z, Liu Y, Yan H. DNA origami with complex curvatures in three-dimensional space. Science. 2011;332(6027):342–346. doi:10.1126/science.1202998.Citation167 Reprinted with permission from AAAS. c, DNA origami pattern. Reprinted by permission from Nature Springer: Nature Nanothechnology, Zhang F, Jiang S, Wu S, et al. Complex wireframe DNA origami nanostructures with multi-arm junction vertices. 2015;10:779. doi:10.1038/nnano.2015.162.Citation168 Copyright (2015). d, From Dietz H, Douglas SM, Shih WM. Folding DNA into twisted and curved nanoscale shapes. Science. 2009;325(5941):725–730. doi:10.1126/science.1174251.Citation169 Reprinted with permission from AAAS.
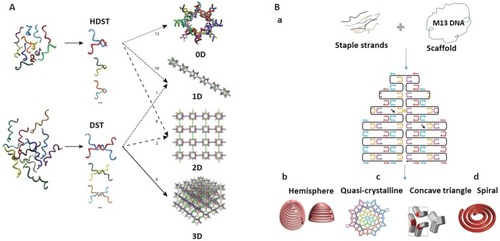
Figure 2 DON for targeted drug delivery. (A) Multiple-armed tetrahedral DNA nanostructure (TDNs) for dual-modality in vivo imaging and targeted cancer therapy. Adapted with permission from Jiang D, Sun Y, Li J, et al. Multiple-armed tetrahedral DNA nanostructures for tumor-targeting, dual-modality in vivo imaging. ACS Appl Mater Interfaces. 2016;8(7):4378–4384. doi:10.1021/ acsami.5b10792.Citation14 Copyright (2016) American Chemical Society. (B) Triangle-shaped DNA origami affords optimum drug internalization for cancer chemotherapy. Adapted with permission from Zhang Q, Jiang Q, Li N, et al. DNA origami as an in vivo drug delivery vehicle for cancer therapy. ACS Nano. 2014;8(7):6633– 6643. doi:10.1021/nn502058j.Citation108 Copyright (2014) American Chemical Society. (C) DON with a different degree of twist and relaxation achieves tunable drug release kinetics. Adapted with approval from Zhao YX, Shaw A, Zeng X, Benson E, Nystrom AM, Hogberg B. DNA origami delivery system for cancer therapy with tunable release properties. ACS Nano. 2012;6(10):8684–8691. doi:10.1021/nn3022662.Citation21 Copyright ACS, https://pubs.acs.org/doi/abs/10.1021%2Fnn3022662. Further permissions related to the material excerpted should be directed to ACS.
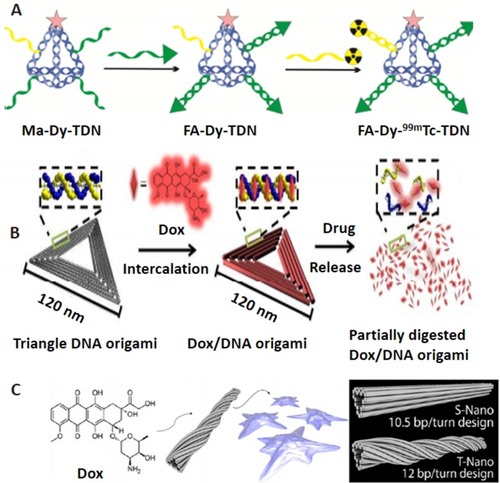
Figure 3 DNA nanocage. (A) Inorganic nanoparticle inside the DNA cage as a hybrid drug delivery system. Reprinted with permission from Zhang C, Li X, Tian C, et al. DNA nanocages swallow gold nanoparticles (AuNPs) to form AuNP@DNA cage core-shell structures. ACS Nano. 2014;8(2):1130–1135. doi:10.1021/nn406039p.Citation20 Copyright (2014) American Chemical Society. (B) Single protein encapsulated in rigid DNA tetrahedron nanocage. Reprinted with permission from Erben CM, Goodman RP, Turberfield AJ. Single-molecule protein encapsulation in a rigid DNA cage. Angew Chem Int Ed Engl. 2006;45(44):7414–7417. doi:10.1002/anie.200603392. John Wiley and Sons.Citation116 (C) Doxorubicin-loaded tumor-penetrating peptide-modified DNA tetrahedron. Reprinted with permission from Xia Z, Wang P, Liu X, et al. Tumor-penetrating peptide-modified DNA tetrahedron for targeting drug delivery. Biochemistry. 2016;55 (9):1326–1331. doi:10.1021/acs.biochem.5b01181.Citation122 Copyright (2016) American Chemical Society. (D) DNA nanosuitcases for encapsulation and conditional release of siRNA. Reprinted with permission from Bujold KE, Hsu JCC, Sleiman HF, Optimized DNA. “Nanosuitcases” for encapsulation and conditional release of siRNA. J Am Chem Soc. 2016;138(42):14030–14038. doi:10.1021/jacs.6b08369.Citation50 Copyright (2016) American Chemical Society. (E) DNA tetrahedron structured probe (TSP) for protein biosensing. Adapted with permission from Pei H, Lu N, Wen Y, et al. A DNA nanostructure-based biomolecular probe carrier platform for electrochemical biosensing. Adv Mater. 2010;22(42):4754–4758. doi:10.1002/adma.201002767. John Wiley and Sons.Citation126
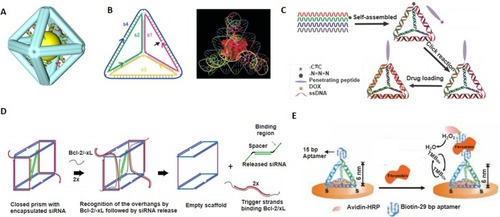
Figure 4 DNA origami arrays and bioreactors. (A) multi-protein decoration of DNA origami structures (arrays) resembling a human face. Adapted with permission from Sacca B, Meyer R, Erkelenz M, et al. Orthogonal protein decoration of DNA origami. Angew Chem Int Ed Engl. 2010;49(49):9378–9383. doi:10.1002/anie.201005931. John Wiley and Sons.Citation130 (B) Assembly steps for the 2D nanocomponent arrays. Adapted with permission from Pinto YY, Le JD, Seeman NC, Musier-Forsyth K, Taton TA, Kiehl RA. Sequence-encoded self-assembly of multiple-nanocomponent arrays by 2D DNA scaffolding. Nano Lett. 2005;5(12):2399–2402. doi:10.1021/nl0515495.Citation132 Copyright (2005) American Chemical Society. (C) 2D streptavidin nanoarrays on rectangular DNA origami surface. Adapted with permission from Kuzuya A, Kimura M, Numajiri K, et al. Precisely programmed and robust 2D streptavidin nanoarrays by using periodical nanometer-scale wells embedded in DNA origami assembly. Chembiochem. 2009;10(11):1811–1815. doi:10.1002/cbic.200900229. John Wiley and Sons.Citation133
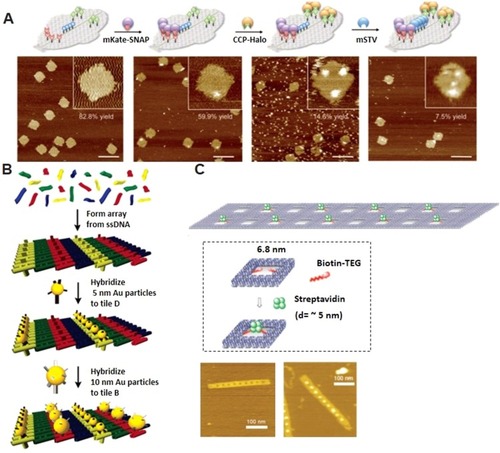
Figure 5 DNA nanoflower preparation and its biomedical applications. (A) miRNA initiated the growth and blooming of DNA nanoflower in nanochannel. Adapted with permission from Shi L, Mu C, Gao T, et al. DNA nanoflower blooms in nanochannels: a new strategy for miRNA detection. Chem Commun. 2018;54(81):11391–11394. doi:10.1039/c8cc05690k. Permission conveyed through Copyright Clearance Center, Inc.Citation135 (B) DNA nanoflowers for multiplexed cellular imaging and traceable targeted drug delivery. Adapted from Hu R, Zhang X, Zhao Z, et al. DNA nanoflowers for multiplexed cellular imaging and traceable targeted drug delivery. Angew Chem Int Ed Engl. 2014;53(23):5821–5826. doi:10.1002/anie.201400323. John Wiley and Sons.Citation10 (C) RCR-based assembly of NFs loaded with chemotherapeutics for targeted drug delivery. Reprinted by permission from Springer Nature: Lv Y, Hu R, Zhu G, et al. Preparation and biomedical applications of programmable and multifunctional DNA nanoflowers. Nat Protoc. 2015;10(10):1508–1524. doi:10.1038/nprot.2015.078.Citation136 Copyright (2015). (D) Synergistic immunotherapy of cancer by DNA-RNA microflowers (iDR-MFs) loaded with tumor neoantigens. Adapted with permission from Zhu G, Mei L, Vishwasrao HD, et al. Intertwining DNA-RNA nanocapsules loaded with tumor neoantigens as synergistic nano-vaccines for cancer immunotherapy. Nat Commun. 2017;8(1):1482. doi:10.1038/s41467-017-01386.Citation68
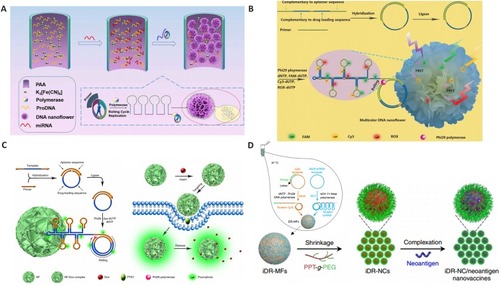
Figure 6 Design and application of DNA dendrimer in biomedicine. (A) DNA dendrimer–streptavidin for efficient signal amplification for biosensing. Adapted with permission from Zhao Y, Hu S, Wang H, et al. DNA dendrimer–streptavidin nanocomplex: an efficient signal amplifier for construction of biosensing platforms. Anal Chem. 2017;89(12):6907–6914. doi:10.1021/acs. analchem.7b01551.Citation140 Copyright (2017) American Chemical Society. (B) Multifunctional DNA dendrimers. Adapted with permission from Qu Y, Yang J, Zhan P, et al. Self-assembled DNA dendrimer nanoparticle for efficient delivery of immunostimulatory CpG motifs. ACS Appl Mater Interfaces. 2017;9(24):20324–20329. doi:10.1021/acsami.7b05890.Citation34 Copyright (2017) American Chemical Society.
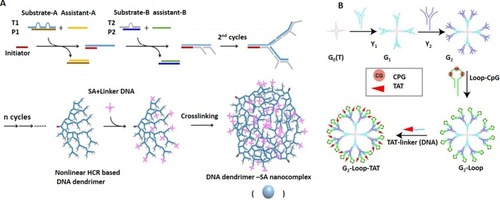
Figure 7 Design and application of DNA hydrogels in biomedicine. (A) Schematic representation of the photo-responsive properties of DNA hydrogels for on-demand cargo release of the payloads. Adapted with permission from Kang H, Liu H, Zhang X, et al. Photoresponsive DNA-cross-linked hydrogels for controllable release and cancer therapy. Langmuir. 2011;27(1):399–408. doi:10.1021/la1037553.Citation146 Copyright (2011) American Chemical Society. (B) Apatmer (EpCAM) binding-mediated catalysis of HCR control gel-sol state of ATP-responsive DNA hydrogel, resulting in cloaking (gel state) and releasing (sol state) of circulating tumor cells. Adapted with permission from Song P, Ye D, Zuo X, et al. DNA hydrogel with aptamer-toeholdbased recognition, cloaking, and decloaking of circulating tumor cells for live cell analysis. Nano Lett. 2017;17(9):5193–5198. doi:10.1021/acs.nanolett.7b01006.Citation61 Copyright (2017) American Chemical Society. (C) Self-assembly of aptamer-based nanohydrogel using Y-shaped building blocks for targeted cancer gene therapy. Adapted with permission from Li J, Zheng C, Cansiz S, et al. Self-assembly of DNA nanohydrogels with controllable size and stimuli-responsive property for targeted gene regulation therapy. J Am Chem Soc. 2015;137(4):1412–1415. doi:10.1021/ja512293f.Citation54 Copyright ACS, https://pubs.acs.org/doi/abs/10.1021%2Fnn3022662. Further permissions related to the material excerpted should be directed to ACS.
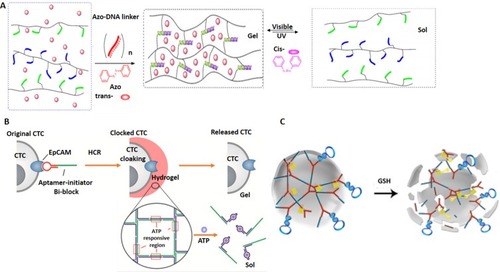
Figure 8 DNA nanoswitch systems as cancer theranostics. (A) Azobenzene-integrated photo controlled drug release from DNA/mesoporous silica. Adapted with permission from YuanQ, ZhangY,Chen T, et al. Photon-manipulated drug release from a mesoporous nanocontainer controlled by azobenzene-modified nucleic acid. ACS Nano. 2012;6(7):6337–6344. doi:10.1021/nn3018365.Citation159 Copyright (2012) American Chemical Society. (B) Redox stimuli-responsive drug release of the PMAA nanohydrogels. Adapted with permission from Pan YJ, Chen YY, Wang DR, et al. Redox/pH dual stimuli-responsive biodegradable nanohydrogels with varying responses to dithiothreitol and glutathione for controlled drug release. Biomaterials. 2012;33(27):6570–6579. doi:10.1016/j.biomaterials.2012.05.062, copyright (2012), with permission from ElsevierCitation160 (C) Aptamer-based DNA nanoswitch for controlled drug release. Adapted with approval of Mo R, Jiang T, Sun W, Gu Z. ATP-responsive DNA-graphene hybrid nanoaggregates for anticancer drug delivery. Biomaterials. 2015;50:67–74. doi:10.1016/j.biomaterials.2015.01.053, copyright (2015) with permission from Elsevier.Citation163
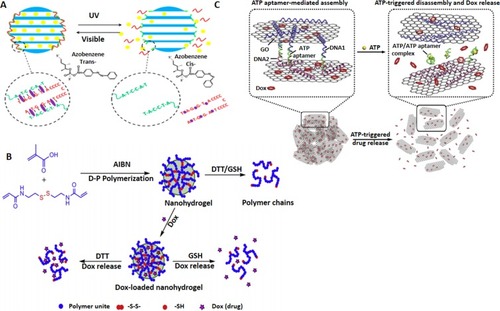