Figures & data
Figure 1 Stromal riboflavin grading scale.Citation54 Grade 0: No green visible; Grade 1: Mild green tint just visible; Grade 2: Substantial green visible; Grade 3: Obvious green color; Grade 4: Bright green appearance; Grade 5: Strong bright green color.
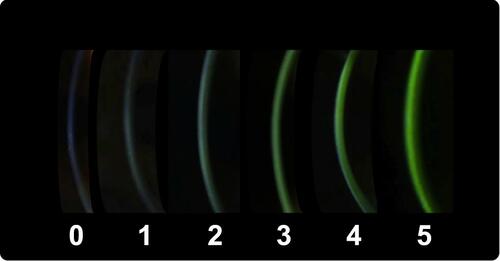
Figure 2 Riboflavin grade vs riboflavin loading time in eyes treated with transepithelial riboflavin formulation with or without NaI in the pilot study (n=4 eyes per formulation).
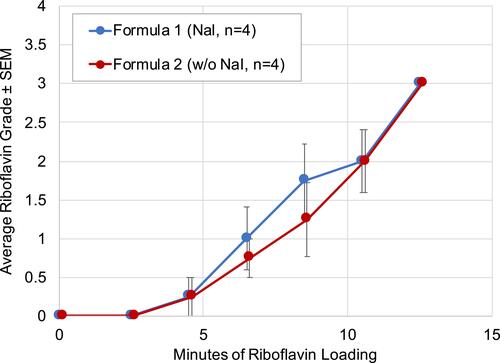
Figure 3 Riboflavin grade vs time throughout UVA exposure in eyes treated with transepithelial riboflavin formulation with or without NaI (n=16 eyes per formulation). Mean riboflavin grade immediately after the riboflavin loading procedure was higher in eyes treated with NaI compared to eyes treated with NaI-free riboflavin formulation. Mean riboflavin grade remained higher in eyes treated with NaI compared to eyes treated with NaI-free riboflavin formulation throughout UVA exposure. Two-way mixed-design ANOVA analysis showed highly significant (p<0.001) differences in overall grade throughout UVA exposure and for change in grade over time. Individual time point P-values are from Sidak post hoc test after ANOVA.
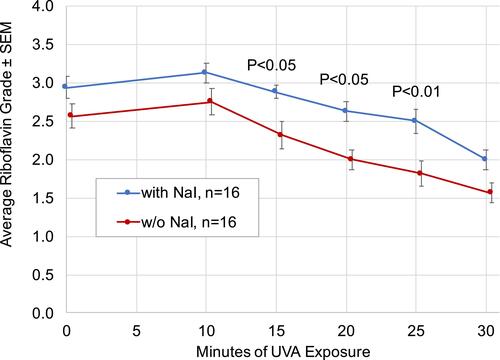
Figure 4 Concentrations of riboflavin and riboflavin 5ʹ-phosphate in the corneal stroma in eyes (n=8 per formulation) treated with transepithelial riboflavin formulation with or without NaI after UVA exposure. (A) Mean riboflavin concentrations. (B) Mean riboflavin 5ʹ-phosphate concentrations; P-values are from unpaired two-tailed t-test. Data shown as mean ± standard error of the mean.
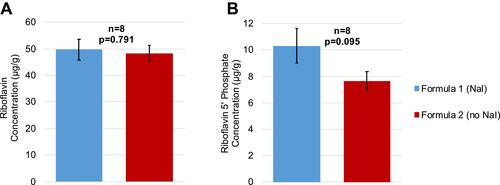
Table 1 Clinical Examination Findings in Eyes Treated with Transepithelial Riboflavin Formulation with or without NaI
Figure 5 Illustration of the two possible reaction pathways for the crosslinking (CXL) photoreaction and hypothesized action of iodide. In low-oxygen conditions, the reaction follows the Type 1 pathway (red arrows), in which excited riboflavin triplets (Riboflavin*3), acting as a cross-linker, reacts with substrate forming radicals (substrate’ and Riboflavin-H’), and then with water to form hydrogen peroxide and derivatives of riboflavin. In the presence of sufficient oxygen, riboflavin reacts directly with oxygen to generate less toxic singlet oxygen (1O2) that cross-links extracellular matrix proteins (Type 2 pathway, green arrows). Sodium iodide (blue dotted arrows) shifts the CXL photoreaction from the toxic Type 1 pathway to favorable Type 2 pathway by quenching excited triplet riboflavin in anaerobic conditions. Further, if Type 1 reactions occur, iodide promotes the immediate conversion of hydrogen peroxide into oxygen and water, providing diatomic oxygen for further crosslinking reactions.
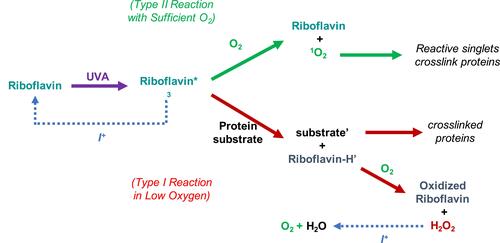